Keywords |
Cordyceps taii; Alkali-soluble polysaccharide; Free radical scavenger; Antioxidant activity; Chemical
characteristics |
List of Abbreviations |
ACP: Alkali-Solube Crude Polysaccharide; ARP: Alkali-
Solube Refined Polysaccharide; APP: Alkali-Soluble Pure
Polysaccharide; ROS: Reactive Oxygen Species; RNS:
Reactive Nitrogen Species; TU: Thiourea; BHA: Butyl
Hydroxyanisole; BHT: Butylated Hydroxytoluene; EDTA:
Ethylenediamine Tetraacetic Acid; DPPH•: 1,1-Diphenyl-2-
picrylhydrazyl free radical, •OH: Hydroxyl Free Radical; •O2-:
Superoxide Anion Free Radical; Fe2+: Ferrous Ions; EC50:
50% Effective Concentration; GC-MS: Gas Chromatography-
Mass Spectrometry; UV: Ultraviolet; FT-IR: Fourier Transform
Infrared Spectroscopy |
Introduction |
Free radicals, generally known as reactive oxygen and nitrogen
species (ROS/RNS), can generate oxidative stress to damage
all cell structures of the living organisms at high
concentrations, and play a major pathological part in the
development of human chronic and degenerative diseases such
as cancer, autoimmune disorders, cirrhosis, arthritis, aging, cardiovascular and neurodegenerative diseases [1-3].
Antioxidants, therefore, play important roles in preventing
these ailments induced by ROS/RNS, and free radicals and
antioxidants have become commonly used terms for these
disease mechanisms in the past twenty years. Also,
antioxidants therapy is receiving much attention around the
world in clinical, as well as the research fields. As we known,
some synthetic antioxidants such as butylated hydroxyanisole
(BHA), butylated hydroxytoluene (BHT) were widely used in
the food and drug industry. Recent studies showed that these
synthetic antioxidants were dangerous towards human health
such as liver damage and carcinogens, and have been forbidden
to use in the food and pharmaceutical industries worldwide [4,5]. Thereby it has resulted in an increasing interest in naturally
occurring alternatives in recent years. Among natural
antioxidantive agents, enzymatic antioxidants are mostly
inactivated in food and drug processing, non-enzymatic
antioxidant agents have received a great deal of attention
accordingly. |
Cordyceps, a well-known and valued traditional Chinese
medicinal macrofungus, is an attractive source for the development of natural antioxidants. Previous studies showed
many Cordyceps fungi such as C. sinensis, C. militaris, C.
jiangxiensis exerted potent antioxidant activities, and
polysaccharides were recognized as major active ingredients
with antioxidant activity in Cordyceps fungi [5-7]. Cordyceps
taii is a folk medicine native to south China [8]. Our group has
demonstrated that C. taii had a wide variety of
pharmacological effects due to its diverse natural active
compounds [9-12]. The water-soluble polysaccharide of C. taii was found to display potent immunomodulatory, and antitumor
activities [8,13]. Furthermore, within our recent screening
program for antioxidative substances from Cordyceps fungi,
the water-soluble polysaccharide of C. taii was found to exert
potent antioxidant and immunoenhancing activities in the
aging mouse model, and was a promising source of natural
antioxidants [14]. To our knowledge, there have been no
reports on the antioxidant activity of the alkali-soluble
polysaccharides of C. taii. Therefore, the antioxidative
potential in vitro and chemistry characteristics of alkali-soluble
polysaccharides of C. taii were developed in the present study. |
Materials and Methods |
Chemicals and reagents |
All chemicals used were of analytical grade. 1, 1-Diphenyl-2-
picrylhydrazyl (DPPH), butylated hydroxytoluene (BHT), and
butyl hydroxyanisole (BHA) from Sigma Inc. (St. Louis, MO,
USA). Ethylenediamine tetraacetic acid (EDTA) and dimethyl
sulphoxide (DMSO) from Invitrogen Inc. (Carlsbad, CA,
USA). DEAE-cellulose-52 gel from Merck (Germany), and
Sephadex G-100 from Amersham Biosciences (Pharmacia,
Sweden). Trichloroacetic acid (TCA), ferrozine, potassium
ferricyanide, ferrous chloride and ascorbic acid from Advanced
Technology and Industrial Co. Ltd (Hongkong, China). Orthophenanthroline
(1, 10-phenanthroline) from Damao Chemical
Reagents Factory (Tianjin, China), and thiourea (TU) from
Kexing biotechnology Co. Ltd. (Shanghai, China). |
Strain, media and mycelia preparation |
The voucher specimen of C. taii GYYA 0601 was deposited at
the Laboratory of Microbial Resource and Drug Discovery,
Center of Translational Medicine of Guizhou Province,
Affiliated Hospital of Zunyi Medical University, China. C. taii was maintained on a slant seed medium (2.5% w/v sucrose,
0.3% soybean, 0.5% yeast extract, 0.2% peptone, 0.3% wheat
bran, 0.1% KH2PO4, 0.05% MgSO4·7H2O, 1.8% agar, and 1 L
of distilled water; initial pH=6.0). The stock culture was
incubated at 28ºC for 15 d, and then stored at 4ºC in a
refrigerator before use. The mycelia of C. taii were prepared as
previously described [14]. Subsequently, the mycelia were
lyophilized and grinded (60 mesh to 100 mesh) for later
experiments. |
Isolation of alkali-soluble polysaccharides |
Dry power of cultured C. taii mycelia (g) was repeatedly
defatted with petroleum ether at room temperature. The residue was got by centrifugation (5000 × g for 10 min), dried at room
temperature, extracted thrice with distilled water in a ratio of
material to water 1:10 (m/v) at 95°C for 2 h, and then
centrifuged at 5000 × g for 10 min. Subsequently, the solid
residue was extracted with 0.5M NaOH solution at 60°C for 2
h, the material/extraction ratio is 1:10 (m/v). After vacuum
filtration, the solid residue was extracted again six times under
the same condition. The combined filtrates were completely
neutralized by 0.5M HCl solution, and then condensed to onefifth
of their total volume using a rotary evaporator under
reduced pressure at 50°C to 55°C. The filtrates were then
precipitated at 4°C for 12 h with ethanol to an 80% final
concentration. The precipitate was washed thrice with 85%
ethanol and acetone then lyophilized in a vacuum, yielding the
alkali-soluble crude polysaccharide of C. taii (ACP, 135 g).
The ACP was stored at 4°C before use. Using the modified
phenol-sulfuric acid method [15], the ratio of ACP fresh
weight to dry cell weight (w/w) was 13.5%, and
polysaccharide content of ACP was 56.1% (w/w). The ACP
was re-dissolved in distilled water to prepare the alkali-soluble
refined polysaccharide (ARP, 18.5 g) as previously described
[14,16]. By the modified phenol-sulfuric acid method [15], the
yield of ARP was determined to be 1.85% (w/w) of the dry cell
weight, and its polysaccharide content was 95.7% (w/w). |
The ARP was successively purified by ion-exchange and gel
filtration column chromatography methods to get alkali-soluble
pure polysaccharide (APP) fractions. The ARP sample (1.0 g)
was dissolved, filtered, and loaded to a column (2.4 cm × 50
cm) of DEAE-cellulose-52. The column was gradiently eluted
with NaCl aqueous solution (0.01 M to 0.15 M), followed by
0.3 M and 0.5M NaOH. Flow rate 2.5 ml/min, 15 ml/tube. This
process was monitored by the modified phenol–sulfuric acid
method [15]. Consequently, three subfractions (ARP-Fr1 to
Fr3) were obtained after DEAE-cellulose chromatography
separation. ARP-Fr2 (398 mg) eluted out of ion-exchange
column with 0.05 M NaCl aqueous solution was further
purified to yield the APP fraction by Sephadex G-100 gel
filtration column chromatography (1.6 cm × 60 cm) with
distilled water elution. The resulting APP fraction was used in
the subsequent chemical analyses. |
Analyses of Chemical properties |
Ultraviolet (UV) spectroscopy of the APP fraction was
detected within 200 nm to 400 nm using a Lambda UV
spectrometer (PerkinElmer, USA). The infrared (IR) spectra of
the APP was determinated within 4000 cm-1 to 400 cm-1 using
a Varian 1000 Fourier-transform Infrared spectroscopy (FT-IR)
instrument (Varian, USA) with KBr pellets. The
monosaccharide composition analysis of APP was performed
using a gas chromatography- mass spectrometry (GC/MS)
method as previously described by our group [14]. |
Assay of DPPH• scavenging |
The modified method as described by Xiao et al. [14] was
applied in this study to assess the scavenging lipid-soluble
DPPH• ability of the alkali-soluble polysaccharide fractions of C. taii. Two milliliter of pH 6.86 phosphate buffer, 2 ml of 0.5
mM fresh DPPH in ethanol solution, and 0.5 ml of the samples
tested at different concentrations were added to a glass tube in
turn. Subsequently, the reaction mixture was shaken
vigorously, and left to stand at room temperature for 30 min in
the dark. The reduction of the DPPH• was measured by
monitoring the absorbance at 520 nm. The radical scavenging
activity was calculated as a percentage of DPPH discoloration
by the following equation: scavenging ability (%)=[1–(As–
Asb)/Ab]×100, where As, Asb, and Ab are the absorbances at
520 nm of the reaction mixture of the sample or antioxidant,
sample blank, and blank, respectively. Here antioxidant
thiourea (TU) was used as the positive control, and the sample
solution without DPPH was used as a sample blank. Ultrapure
water was used as the blank control without samples or
antioxidant. All the tests were performed in triplicate. |
Assay of •OH scavenging |
The modified ortho-phenanthroline method was applied to
detect the scavenging ability on •OH produced by Fenton
reaction of the alkali-soluble polysaccharide fractions of C. taii [14]. In this study, phosphate buffer (4 ml, pH 7.4),
orthophenanthroline in ethanol (1.5 ml, 5 mM) and FeSO4 (1
ml, 7.5 mM) were immediately mixed. The samples (1 ml) at
different concentrations, ultrapure water (1.5 ml), and
hydrogen peroxide (1 ml, 1% v/v) were then added to the
mixture in sequence. After incubating at 37°C for 60 min, the
absorbance change of reaction mixture caused by the color
change of iron-orthophenanthroline was measured at 510 nm.
The antioxidant TU was used as the positive control, the
ultrapure water in place of sample and antioxidant was used as
damage control (the control of the •OH generation system) and
the ultrapure water was used as the blank without sample,
antioxidant and hydrogen peroxide. All the tests were
performed in triplicate. The •OH scavenging activity was
calculated using the following equation: Scavenging ability
(%)=[(As-Ao)/(Ab-Ao)] × 100. Where, As, Ao and Ab are the
absorbance at 510 nm of reaction mixture of the sample or
antioxidants, damage control and blank, respectively. |
Assay of •O2- scavenging |
2,4-Iodiphenyl-3,4-nitrophenyl-5-phenyltetrazolium chloride
(NBT) method was employed to measure the scavenging •O2- activity of the alkali-soluble polysaccharide fractions of C. taii.
Here an antioxidant kit was used as previously reported by
Xiao et al. [5]. The •O2- generated by the xanthine - xanthine
oxidase system reacted with the NBT to form amethyst
formazan. The absorbance of formazan in the reaction system
measured at 550 nm was used to reflect indirectly the presence
of the •O2-. Here the production of formazan is inversely
related to the •O2- scavenging ability of the samples tested.
Therefore, the final results were expressed as the inhibition
degree of formazan production. BHA was used as the positive
control and ultrapure water was used in the place of samples or
antioxidant as blank. All the tests were performed in triplicate.
The percentage inhibition of the •O2- was calculated as (1-As/Ab) × 100. Where As, and Ab are the absorbance of the
reaction mixture of the sample or antioxidant, and blank at 550
nm, respectively. |
Assay of reducing ability |
The modified Prussian blue method as described by Xiao et al.
[5] was employed to assess the Fe3+ to Fe2+ transformation
ability for the total antioxidant potential of the alkali-soluble
polysaccharide fractions of C. taii. The samples (1 ml) at
different concentration, phosphate buffer (2.5 ml, pH 6.6), and
K3Fe(CN)6 (2.5 ml, 1%, m/v) were mixed in a glass tube, and
then incubated at 50°C for 20 min. The mixture was added into
TCA (2.5 ml, 10% m/v), followed by centrifugation at 1000 ×
g for 10 min. The supernatant (2.5 ml) was diluted with
isometric ultrapure water, and was then reacted for 10 min by
the addition of 0.1% fresh ferric chloride (2.5 ml). Finally, the
reaction mixture was cooled to room temperature, and the
absorbance was measured at 700 nm. The antioxidant BHA
was used as the positive control. The reference solution was
prepared as stated earlier and was used as the blank, but
contained ultrapure water instead of the samples or BHA. The
total antioxidant ability was expressed as the absorbance value
at 700 nm, and higher absorbance value of the reaction mixture
indicates greater antioxidant activity. The reducing ability was
calculated as follows: A=As-Ab, Where, Ab is the absorbance
of the blank, and As is the absorbance of the polysaccharide
fractions of the sample or antioxidants at 700 nm. Three
replicates were carried out for all tests |
Assay of Fe2+ chelating activity |
The chelating activity of the alkali-soluble polysaccharide
fractions of C. taii on ferrous ion was measured as reported by
Xiao et al. [5]. Samples (0.5 ml) at different concentration,
ultrapure water (1.8 ml), FeCl2 (0.05 ml, 2 mM) and ferrozine
(0.1 ml, 5 mM) were mixed in a glass tube. Here ferrozine
reacted with the divalent iron to form stable magenta complex
that was very soluble in water. After 20 min at room
temperature, the absorbance of the Fe2+- ferrozine complex in
the reaction system was measured at 562 nm. EDTA was used
as positive control, and ultrapure water was used in place of the
sample or EDTA as blank. Three replicates were carried out for
all tests The chelating activity of the polysaccharide fraction on
Fe2+ was calculated as chelating rate (%)=(Ab-As)/Ab × 100.
Where, Ab is the absorbance of the blank without sample or
EDTA, and As is the absorbance in the presence of the sample
or EDTA. |
Statistical analysis |
The experimental results were processed by SPSS 13.0 (SPSS
Inc.). The data were analyzed by one-way analysis of variance
(ANOVA), and were expressed as mean ± standard deviation
of triplicate determinations. Dunnett’s t-test was used to
compare the differences between the treated groups and control
groups and differences were regarded as significant at p<0.05. |
Results |
Scavenging the DPPH• ability of alkali-soluble
polysaccharide fractions |
As shown in Figure 1A, two different polysaccharide fractions
from the cultured C. taii showed moderate activities against the
DPPH radicals at most of the tested doses, and their
scavenging abilities were increased with increased doses
ranging from 0.5 mg/ml to 8.0 mg/ml in a dose-dependent
manner. Among the tested fractions, the ACP fraction showed
stronger activity than ARP fraction at the same doses.
However, the scavenging activities of the positive control TU
were stronger than that of the polysaccharide samples at all the
tested doses. |
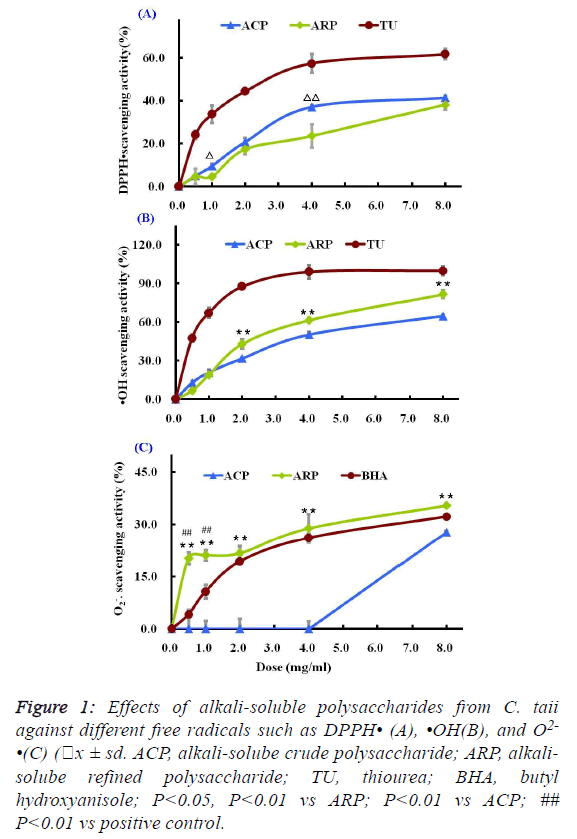 |
Scavenging the •OH ability of alkali-soluble
polysaccharide fractions |
The scavenging effects of two different polysaccharide
fractions on hydroxyl radicals were shown in Figure 1B. Both
ACP and ARP all exhibited potent scavenging abilities towards
hydroxyl radicals in a dose-dependent manner at all the tested
doses. Especially ARP had more significant scavenging
activity against the hydroxyl radicals than ACP at doses ranging from 2.0 mg/ml to 8.0 mg/ml. The scavenging rate of
ARP reached 81.73 ± 1.89% at a high dose of 8.0 mg/ml.
However, their scavenging abilities to hydroxyl radicals were
lower than that of the radical scavenger TU. TU at a dose of 4
mg/ml showed a dramatic inhibition of hydroxyl radical
(99.09% ± 5.26%). |
Scavenging the O2-• ability of alkali-soluble
polysaccharide fractions |
The superoxide anion radicals scavenging activities of the
different polysaccharide fractions from the cultured C. taii were given in Figure 1C. The ACP fraction at doses ranging
from 0.5 mg/ml to 8.0 mg/ml showed no activities against the
superoxide anion radicals. However, the ARP fraction could
more significantly scavenge the superoxide anion radicals in
comparison with the radical scavenger BHA at all the tested
doses, and also appeared a dose-dependence response. |
Effect of alkali-soluble polysaccharide fractions on
the reducing power |
The Fe3+-Fe2+ transformation assay is a standardized
antioxidant capacity method. In this assay, the presence of
reductant in the antioxidant sample can cause the reduction of
the Fe3+/ferricyanide complex to the Fe2+/ferrous form, so the
reducing power of the antioxidant sample can be monitored by
measuring the formation of Prussian blue at a wavelength of
700 nm. |
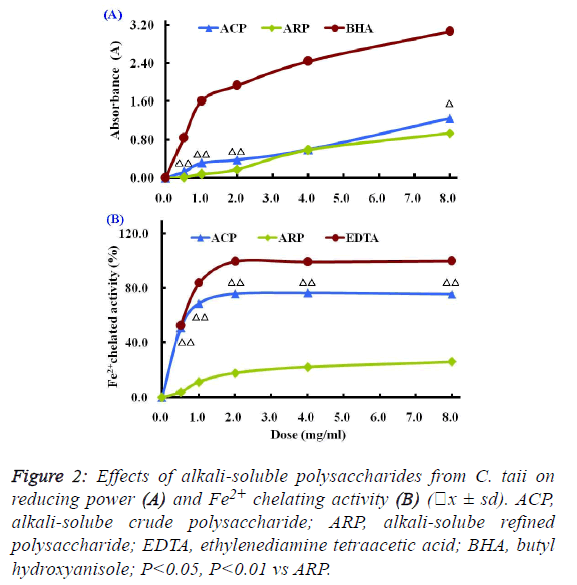 |
Compared with the positive control BHA, the reducing powers
of different polysaccharide fractions from C. taii presented
moderate abilities as shown in Figure 2A. Also, the reduction
potential of ACP and ARP fractions exhibited a dosedependent
manner within the concentration range of 0.5 mg/ml
to 8.0 mg/ml. |
Effect of alkali-soluble polysaccharide fractions on
the Fe2+ chelating activity |
The Fe2+ chelating activities of the different polysaccharide
fractions from the cultured C. taii are shown in Figure 2B.
Similar to the above results of antioxidant activity, the
chelating abilities of the polysaccharide fractions ACP and
ARP exhibited in dose-dependent manner at all the tested
doses of 0.5 mg/ml to 8.0 mg/ml, in particular ACP exerted a
high Fe2+ chelating potential of greater than 70% at doses up to
1.0 mg/ml. Furthermore, like the positive control EDTA, the
chelating activities of ACP sharply increased with increased
dose from 0 mg/ml to 1mg/ml, and reached a plateau at a dose
of 2.0 mg/ml. However, here ARP only gave a markedly lower
chelating activity at all the tested doses compared with EDTA. |
50% effective concentration (EC50) values for
antioxidant properties of alkali-soluble
polysaccharide fractions |
The antioxidant properties were normalized and expressed as
EC50 value for the comparison among different samples, and
the EC50 values of ACP and ARP were summarized in Table 1.
In terms of the EC50 values, there existed certain differences of
the antioxidant properties between ACP and ARP. For
example, the scavenging abilities against •OH and O2-• of ARP
fraction presented about 2-fold stronger than that of ACP
fraction. |
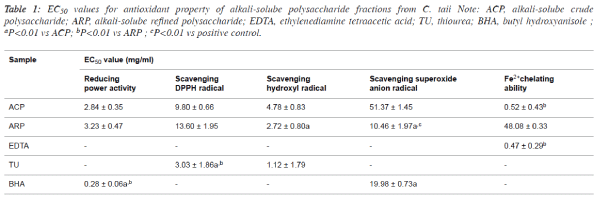 |
However, the reducing power and scavenging DPPH• capacity
of ARP were slightly lower than that of ACP. Interestingly,
ACP displayed potent Fe2+ chelating activities with the low
EC50 value at 0.52 ± 0.43 mg/ml, which was approximately
similar to the most frequently chelating agent EDTA. |
Chemical properties and composition of ARP from C.
taii |
As described above, the polysaccharide fraction of cultured C.
taii displayed a potential source for the development of natural
antioxidants. Therefore, it is necessary to further elucidate its
chemical properties. Here ARP is a fine alkali-soluble
polysaccharide fraction from ACP through depigmentation,
deproteinization, dialyzation, and ethanol precipitation. After
fractionation on DEAE-cellulose-52, ARP-Fr1 (278 mg), ARPFr2
(398 mg), and ARP-Fr3 (80 mg) were isolated by 0.01 M,
0.05 M and 0.1M NaCl elution, respectively (Figure 3A).
Subsequently, the ARP-Fr2 fraction was further purified by gel
filtration chromatography on a Sephadex G-100 column with
distilled water elution. As a result, a major APP fraction was
acquired. The homogeneity of APP fraction was then
demonstrated by the following analysis. The APP fraction was
eluted again with distilled water on the Sephadex G-100 gel
column, and could only yield a single peak according to the
elution profile as shown in Figure 3B. Furthermore, APP, as a
subfraction of ARP-Fr2, didn’t like the ACP and ARP fractions, and appeared no specific absorption at 260 nm and/or
280 nm by the scanning analysis of full wavelength UV
(Figure 4A). It implied that the APP fraction had no proteins
and nucleic acids accordingly. As shown in Figure 4B, the IR
absorption spectrum of APP presented main absorption bands
at 3420, 3158, 1637, 1401, 1223, 1043, and 809 cm-1. The
largest absorption band at 3420 and 3158 cm-1 exhibited broad
and intense stretching, which were assigned to hydroxyl groups
(O-H or N-H and C-H). The band between 950 and 1222
cm-1 were mostly attributed to C–O–C and C–O–H linkages.
The stretching peak at 1042 cm-1 was suggestive of a C–O
bond. The band at 1637 cm-1 and 1401 cm-1 can be attributed
to water bound to the polysaccharide molecules [17]. The small
absorption band at 809 cm-1 suggested that APP contained α-
type glycosidic linkages in its structure [14]. Further
investigation showed that APP was composed of only glucose,
mannose, and galactose by the GC-MS analysis (Figure 5), and
theirs molar ratios were 1.14: 1.66: 1.0 with serials α-(1,4)
glycosidic bond by iodine reaction. In addition, mannose may
be the backbone of the structure of APP on the basis of their
molar ratios. |
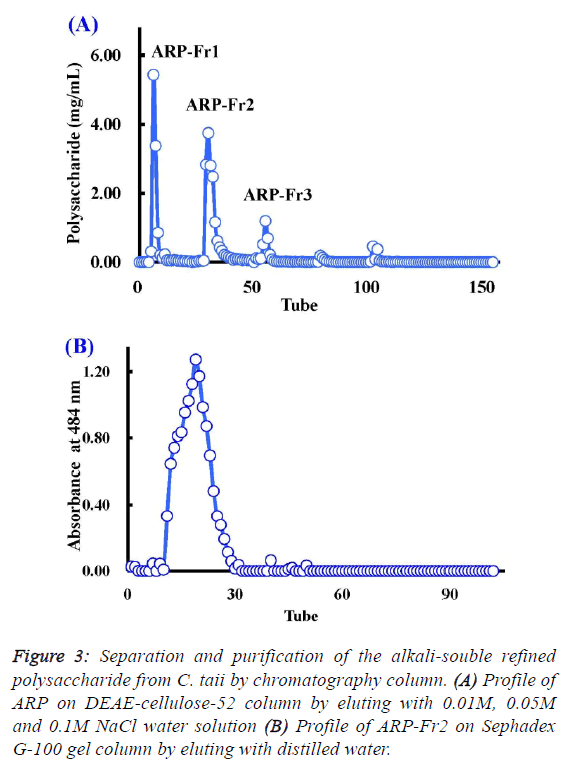 |
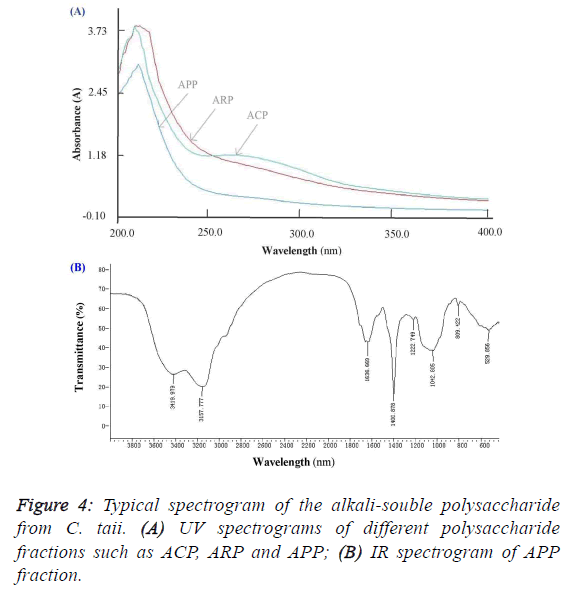 |
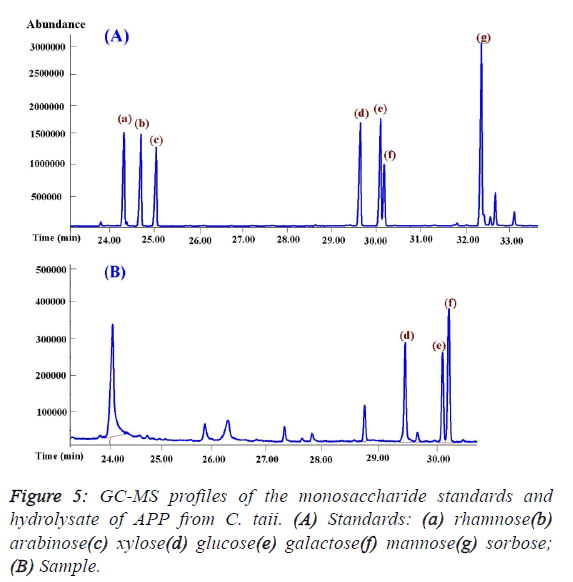 |
Discussion |
Many previous studies only described the antioxidant activites
of crude polysaccharide and/or aqueous extract of Cordyceps species [18-20]. Therefore, it was essential to further reveal the
antioxidant ingredients for the development of natural
antioxidant agents. In this present study, we demonstrated that
both the ACP and the ARP of cultured C. taii could exert
moderate antioxidant potentials. However, there was a
significant difference between both samples towards different
antioxidant assays. For example, the antioxidant properties of
ACP in the range of 0.5-8 mg/ml, including the DPPH•
scavenging ability, the reducing power, and Fe2+ chealting
ability, were superior to that of the ARP. Surprisingly, here the
ACP exhibited a potent chealting activity with an
approximately EC50 value (0.52 mg/ml) compared with the
most frequently chelating agent EDTA (0.47 mg/ml). While the
ARP displayed more outstanding scavenging potencies on •OH
and •O2- than the ACP in this study. As described in materials
and methods section, the ARP was consisted of more than 95%
polysaccharide, and 56% polysaccharide for the ACP only.
Thus polysaccharides were major active ingredients against
•OH and •O2-. Furthermore, many investigations reported that
the purified polysaccharides from other Cordyceps species
mainly exhibited potent scavenging free radicals such hydroxyl
radical [21-23]. Partial antioxidant properties of the ACP,
especially in the chealting activity, had more significant
activities compared with the ARP, the non-polysaccharide
unknown antioxidant compounds were interesting for further
investigation accordingly. In comparison with the previous
investigation by our group 14, there had approximately
antioxidant potentials between the alkali- and water-souble
crude polysaccharide fractions in cultured C. taii. However,
there exist distinguished differences among their refined
polysaccharides, of which the antioxidative abilities such as scavenging DPPH•, and •OH, and reducing power of the alkalisouble
refined polysaccharide were least 2-fold than that of the
water-souble refined polysaccharide. In addition, the watersouble
crude polysaccharide possessed stronger antioxidative
abilities than its refined polysaccharide at all the tested
parameters, including scavenging abilities on DPPH•, •OH and
•O2-, Fe2+ chelating and reducing power [14]. As above, the
water-souble crude polysaccharide attenuated its antioxidant
abilities after purification, the antioxidant components of the
water-souble crude polysaccharide should involve in nonpolysaccharide
antioxidant ingredients such as polyphenols,
flavonoids, and protein. For example, the water-souble crude
polysaccharides of Cordyceps sinensis and Cordyceps militaris contained polyphenolic and flavonoid compounds, which may
in part be responsible for their antioxidant activities [19].
Therefore, these findings suggested that the alkali-souble
polysaccharide was major antioxidant active fraction among
different polysaccharides of C. taii, and its antioxidant
protective effect was a result of its free radicals scavenging
ability. In addition, the scavenging DPPH• abilities of ACP and
ARP with EC values of 9.8 mg/ml, and 13.6 mg/ml were
significantly inferior to that of the positive control TU
(EC50=3.03 mg/ml) in this study. Likewise the aqueous
polysaccharide of C. taii and C. jiangxiensis also showed weak
sensitivities towards to DPPH• [5,14]. These antioxidant
substances, therefore, presented a possible selective difference
against free radical. For this reason, the DPPH is a stable
hydrophobic free radical that possibly interferes with the
scavenging abilities of water-souble antioxidants. Previous
study also demonstrated that the water extracts of both C.
militaris and C. sinensis displayed more sensitive to free
radicals in hydrophilic system than in hydrophobic system
[19]. |
In the further chemical composition analysis of the
polysaccharide, the APP fraction from the ARP fraction of C.
taii was identified as a heteropolysaccharide fraction composed
of glucose, galactose and mannose. The result was in
agreement with the water-souble polysaccharide of C. taii. However, the molar ratios of monosaccharide compositions,
and other chemical properties were different, e.g. glucose was
its backbone [14]. As a result, their antioxidant abilities
presented also evident differences as the above described.
Actually, many polysaccharides with antioxidant activity from Cordyceps fungi were composed of glucose, galactose and
mannose. For instance, Li et al. reported that a polysaccharide
being composed of glucose, mannose, and galactose in C.
sinensis showed antioxidant activity [24]. Yu et al.
demonstrated that P70-1 polysaccharide fraction isolated from
the water-souble crude polysaccharide of cultured C. militaris was also composed of glucose, mannose, and galactose, and
possessed potent hydroxyl radical-scavenging activity with an
EC50 value of 0.548 mg/ml [22]. Therefore, the
heteropolysaccharides composed of glucose, mannose, and
galactose in Cordyceps fungi are a potential source for the
development of natural antioxidant agent. |
Conclusion |
In summary, our results suggest that the alkali-souble
polysaccharide has a moderate antioxidant potential compared
with the synthetic antioxidant agents, and its antioxidant
protective effect is mainly a result of its free radical scavenging
ability. Therefore, it is a potential source for the development
of natural antioxidant agents. Interestingly, the alkali-souble
crude polysaccharide shows a surprising chelating activity due
to some unidentified non-polysaccharide ingredients. The
further work is still necessary to reveal additional chemicals
and their structure-function relationship accordingly. |
Competing interests |
The authors declare that they have no competing interests. |
Acknowledgments |
The authors are grateful to financial supports from the National
Natural Science Foundation of China (No. 81260278),
Guizhou High-Level Innovative Talent Support Program (No.
QKH-RC-20154028), and Program for Innovative Research
Team in Guizhou Province (No. QKH-RCTD-20134035). |
References |
- Valko M, Leibfritz D, Moncol J, Cronin MT, Mazur M, Telser J. Free radicals and antioxidants in normal physiological functions and human disease. Int J Biochem Cell Biol. 2007; 39: 44-84.
- Pham-Huy LA, He H, Pham-Huy C. Free radicals, antioxidants in disease and health. Int J Biomed Sci. 2008; 4: 89-96.
- Uttara B, Singh AV, Zamboni P, Mahajan RT. Oxidative stress and neurodegenerative diseases: A review of upstream and downstream antioxidant therapeutic options. Curr Neuropharmacol. 2009; 7: 65-74.
- Xiao JH, Xiao DM, Sun ZH, Xiao Y, Zhong JJ. Antioxidative potential of polysaccharide fractions produced from traditional Chinese medicinal macrofungus Cordyceps jiangxiensis in vitro. Afr J Biotechnol. 2011; 10: 6607-6615.
- Lobo V, Patil A, Phatak A, Chandra N. Free radicals, antioxidants and functional foods: Impact on human health. Pharmacogn Rev. 2010; 4: 118-126.
- Leung PH, Zhao S, Ho KP, Wu JY. Chemical properties and antioxidant activity of exopolysaccharides from mycelial culture of Cordyceps sinensis fungus Cs-HK1. Food Chem. 2009; 114: 1251-1256.
- Yu HM, Wang BS, Huang SC, Duh PD. Comparison of protective effects between cultured Cordyceps militaris and natural Cordyceps sinensis against oxidative damage. J Agric Food Chem. 2006; 54: 3132-3138.
- Liang ZQ. Flora Fungorum Sinicorum, Cordyceps, Science Press, Beijing, China, 32 edition; 2007.
- Xiao JH, Xiao DM, Sun ZH, Xiong Q, Liang ZQ, Zhong JJ. Chemical compositions and antimicrobial property of three edible and medicinal Cordyceps species. J Food Agric Environ. 2009; 7: 91-100.
- Liu RM, Zhang XJ, Liang GY, Yang YF, Zhong JJ, Xiao JH.Antitumor and antimetastatic activities of chloroform extract of medicinal mushroom Cordyceps taii in mouse models. BMC Complem Altern Med. 2015; 15: 216.
- Li XG, Pan WD, Luo HY, Liu RU, Xiao JH, Zhong JJ. New cytochalasins from medicinal marcofungus Cordyceps taii and their inhibitory activities against human cancer cells. Bioorg Med Chem Lett. 2015; 25: 1823-1826
- Liu RM, Zhang XJ, Li XF, Xiao JH, Zhong JJ. Simple and rapid method for simultaneous determination of eleven nucleosides and nucleobases in medicinal macrofungus Cordcyeps taii. Lat Am J Pharm. 2015; 34: 283-290.
- Xiao JH, Xiao DM, Xiong Q, Liang ZQ, Zhong JJ. Nutritional requirements for the hyperproduction of bioactive exopolysaccharides by submerged fermentation of the edible medicinal fungus Cordyceps taii. Biochem Eng J. 2010; 49: 241-249.
- Xiao JH, Xiao DM, Chen DX, Xiao Y, Liang ZQ, Zhong JJ. Polysaccharides from the medicinal mushroom Cordyceps taii show antioxidant and immunoenhancing activities in a D-galactose-induced aging mouse model. Evid-Based Compl Alt Med. 2012 273435.
- Xiao JH. A rapid method for the determination of polysaccharide in Cordyceps jiangxiensis. J Chinese Med Mat. 2008, 31: 689-692.
- Xiao JH, Liang ZQ, Liu AY Chen DX, Xiao Y, Liu JW, Wan WH. Immunosuppressive activity of polysaccharides from Cordyceps gunnii mycelia in mice in vivo/vitro. J Food Agric Environ. 2004; 2: 69-73.
- Yan JK, Li L, Wang ZM, Wu JY. Structural elucidation of an exopolysaccharide from mycelial fermentation of a Tolypocladium sp. fungus isolated from wild Cordyceps sinensis. Carbohydr Polym. 2010; 79: 125-130.
- Zhan Y, Dong CH, Yao YJ. Antioxidant activities of aqueous extract from cultivated fruit-bodies of Cordyceps militaris (L.) Link in vitro. J Integr Plant Biol. 2006; 48: 1365-1370.
- Yu HM, Wang BS, Huang SC, Duh PD. Comparison of protective effects between cultured Cordyceps militaris and natural Cordyceps sinensis against oxidative damage. J Agric Food Chem. 2006; 54: 3132-3138.
- Wang M, Meng XY, Yang RL, Qin T, Wang XY, Zhang KY, Fei CZ, Li Y, Hu YL, Xue FQ. Cordyceps militaris polysaccharides can enhance the immunity and antioxidation activity in immunosuppressed mice. Carbohydr Polym. 2012; 89: 461-466
- Yu R, Yin Y, Yang W, Ma W, Yang L, Chen X, Zhang Z, Ye B, Song L. Structural elucidation and biological activity of a novel polysaccharide by alkaline extraction from cultured Cordyceps militaris. Carbohydrate Polymers. 2009; 75: 166-171
- Yu R, Yang W, Song L, Yan C, Zhang Z, Zhao Y. Structural characterization and antioxidant activity of a polysaccharide from the fruiting bodies of cultured Cordyceps militaris. Carbohydr Polym. 2007; 70: 430-436.
- Wang Y, Wang M, Ling Y, Fan W, Wang Y, Yin H. Structural determination and antioxidant activity of a polysaccharide from the fruiting bodies of cultured Cordyceps sinensis. Am. J. Chin. Med. 2009; 37: 977-989.
- Li SP, Zhao KJ, Ji ZN, Song ZH, Dong TT, Lo CK, Cheung JKH, Zhu SQ, Tsim KWK. A polysaccharide isolated from Cordyceps sinensis, a traditional Chinese medicine, protects PC12 cells against hydrogen peroxide-induced injury. Life Sci. 2003; 73: 2503-2513.
|