Keywords |
Kaempferol, Phytoestrogen, Flavonoids, Fracture healing, Mouse tibia fracture model. |
Introduction |
Fracture healing is a physiological process that relates to
bone regeneration. It is a dynamic process coordinated
by multiple cell activities including cell proliferation,
differentiation, migration, angiogenesis, and remodeling.
Fracture healing is divided into four overlapping biological
stages namely, the early inflammatory response, soft callus
formation, hard callus formation and initial bony union
followed by bone remodeling process. Early inflammatory
stage occurs immediately after a fracture with hematoma
formation and repair cells recruitment initiated by a cascade
of growth factors and cytokines secretion. Secreted factors
facilitate the recruitment of mesenchymal stem cells
(MSCs) which then start differentiating into specialized
cells to build new bone tissue (osteoblasts) and new
cartilage (chondroblasts). The second stage is characterized
by the formation of soft callus, carried out by chondrocytes
and fibroblasts. Both of these cells build a semi-rigid soft
callus for providing mechanical support to the fracture and
at the same time act as a template for the bony callus. The
third stage is of hard callus formation in which osteoblasts
have a major role to play and are responsible for initial
woven bone matrix. Henceforth, the stage is regarded as the primary bone formation event and represents the most
active stage of osteogenesis. The final stage is a remodeling
phase of action of osteoblast (bone forming cells) and
osteoclast (bone resorption cells) to remodel callus into
stronger lamellar bone [1] and is often termed as secondary
bone formation. However, once in a while fracture healing
may be delayed or impaired by some complex clinical
conditions, including osteoporosis, resulting in tardy or
permanent failure of healing (nonunion) as well as survival
of injures. During such state, normal regenerative process
is generally compromised and patients require quite a few
months to achieve an adequate mechanical competence to
support normal physiological loads. |
Therefore, therapies focusing on improving quality and
the rate of repair of fracture healing are required. The
search of compounds that could efficiently improve repair
process under less ideal conditions would be of significant
importance for both social and economic benefits [2, 3, 4]. |
In the quest of above said objectives, several therapeutic
agents have been intensively reported to improve fracture
healing including estrogen hormone, growth factors
(bone morphogenetic proteins, BMPs) and osteoporosis
drugs (parathyroid hormone, bisphosphates) [2, 5, 6, 7]. However, there are some significant limitations of using
current available agents. Use of estrogen therapy may
increase risk of hormone-sensitive cancers while adverse
effect of delayed remodeling process is being found
associated with the treatment of bisphosphates [8], and
there are several concerns on delivery system, bioactivity
and limitation of the case treatment related to BMPs [9,
10]. Until now, no systematic agent has been registered
with the implications of improvement of fracture healing.
Moreover, considering the drawbacks associated with
above mentioned therapies, new safe and bioactive agents
are urgently required to manage bone fracture healing
process with minimal side effects [11]. |
Owing to the absence of clinical side effects and cheaper
alternatives to available therapies, traditional integrative
medicine has also been considered on improving
effectiveness of fracture healing both in animal models as
well at clinical level [12, 13, 14]. Flavonoids, a type of
natural compounds that are found in many kinds of fruits,
vegetables, tea and traditional herb medicine have been
reported to exert their effects on reducing bone loss and
promoting bone formation. Recently, scientists observed
positive effect of phytoestrogens and formononectin on
early fracture healing [15, 16]. The flavonol Kaempferol
is another popular flavonoid which is present in a variety
of vegetables and fruits and has been reported to have
protective effect against bone loss and increase bone
strength both in vitro and in vivo models [17, 18, 19]. It
is suggested that Kaempferol may be a promising agent
for treatment of postmenopausal osteoporosis [20].
Kaempferol is known to possess anti-osteoclastogenic
effects in vitro such as induction of osteoclast apoptosis
and inhibition of osteoclastic bone resorption [21, 22].
Moreover, Kaempferol has been shown to promote
osteoblast function ex vivo, induce bone formation
in vivo [23] and protect osteoblast from damage by
oxidative stress [24]. In addition, Kaempferol has been
demonstrated to induce osteoblastic differentiation in
rat primary osteoblasts [25], murine mesenchymal cell
line and human primary mesenchymal stem cells [26].
Chondrogenic differentiation and formation of cartilage
nodules can also be stimulated by treatment of Kaempferol
in pre-chondrogenic, ATDC5 cell line [27]. |
However, there is no study available for the effect of
Kaempferol on fracture healing process in the literature.
Therefore, we aimed to evaluate the effect of Kaempferol
on fracture healing process using murine tibial fracture
model produced by standardized equipment. |
Materials and Methods |
Generation of Standardized Fracture of Mouse Tibia Shaft |
Eight-week-old outbred ICR (Institute of Cancer Research)
Swiss mice weighing 29.0 ~ 30.5 g were purchased from
Taconic Inc., South Korea. All the experimental studies
on animals were approved by the institutional ethical
committee on animal use for research and education at Hallym University (Hallym 2013-2092). A standardized
fracture was generated as previously reported by Hiltunen
et al., with minor modifications [28, 29]. Mice were
anesthetized with intraperitoneal injection of pentobarbital
(50 mg/kg) before surgery. A short incision was made on
the dorsolateral side of the thigh and was extended over
the knee region and a longitudinal incision was made in
the patellar tendon. A small 0.5 mm diameter hole was
drilled above the tibia tuberosity. Intramedullary fixation
was made to stabilize fracture by introducing an Anticorro
insect pin into the intramedullary canal of the tibia. The
wound was closed by needle, and the same procedure was
carried out on the contralateral tibia and on tibiae of all
mice used for the experiment. After that, the animal was
placed supine under the apparatus fracture apparatus as
shown in Figure 1A and the anteromedial diaphysis of the
tibia, in the region of the lower midshaft, was exposed to
the blunt blade. A weight of 220 g was dropped from a
height of 195 mm to produce a closed fracture. Fractures
were made in both tibiae of each mouse. |
Administration of Kaempferol to Standardized Fracture
Model |
Twenty male ICR mice were randomly divided into four
groups (n=5/group) and bilateral fractures were created
as described above. The other 5 mice were exposed to a
sham surgical procedure without fracture. From the next
day after making fractures, Kaempferol (Sigma) in the
form of gavage (doses of 0.2, 0.5, 5.0 mg/kg body weight
in 20% ethanol) was orally administered every day for 21
days. The vehicle group was given 20% ethanol instead.
After 21 days, all mice were sacrificed under anesthesia
by cervical dislocation and both tibiae were collected for
analysis. |
Radiographic Imaging and Histology |
Mice tibiae obtained after 21 days were examined using X-ray system for radiographic appearance. For
microscopic analysis, the tissue at the fracture site was
harvested, fixed in 4% paraformaldehyde, decalcified
in 20% ethylene-di-amine tetra-acetic acid (EDTA; pH
7.4), and was embedded in paraffin. Sections with 5μm
thickness were prepared and stained with hematoxylin and
eosin (H&E). All five mice in each group were used for
histological analyses. |
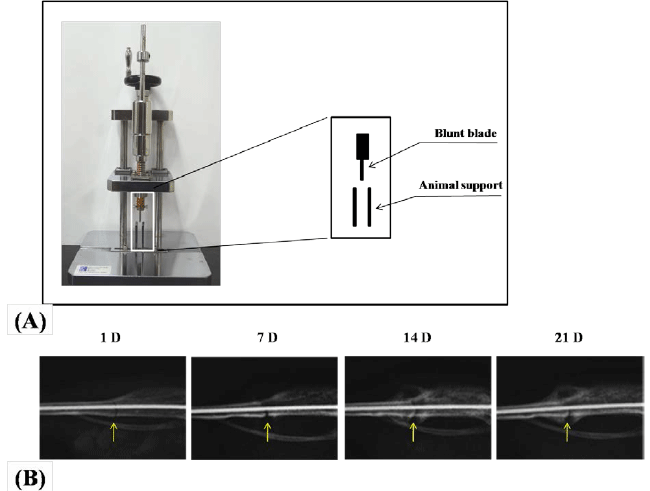 |
Figure 1. Establishment of mouse tibia fracture model.
Controlled fracture machine used to create a standardized
fracture pattern in mouse tibia (A). Radiographical analysis
of fracture healing process in mice tibia, which was stabilized
by an intramedullary pin (B). Radiographs were taken at 1
day (1D), 7 days (7D), 14 days (14D) and 21 days (21D) after
fracture. Arrows indicate fracture sites. |
Measurement of Callus Diameter |
Callus dimension was determined at 21 days after
euthanization of mice. The fracture site of the tibia
was identified by direct dissection and both tibiae were
collected. The dimension of the callus was measured in
two dimensions; anterior-posterior and lateral-medial
using a pocket thickness gage No.7309 (Mitutoyo corp.,
Japan). The average diameter of the fracture callus was
then calculated from these two measurements. |
Statistical Analysis |
Statistical differences were analyzed by Graphpad Prism
5.0 (San Diego, CA) and evaluated by two-tailed Student’s
t-test. A p-value less than 0.05 were considered statistically
significant. |
Results |
Radiologic Characterization of Callus Formation
During Healing Process |
In our study, mice fractures were made transverse or
slightly oblique in the lower midshaft of the tibiae by
using a standardized fracture generation method and
intramedullary fixation was made to facilitate bone healing
(materials and methods, and Figure 1A). Radiographic
examination revealed good alignment of the fractured tibiae
after fixation. Radiographic images taken on day 1, day 7,
day 14 and day 21 indicated continuous stages of healing
process in mice tibia fractures, in which the external callus
formation surrounding the fracture site was noticeably
observed. As shown in Figure 1B, a sharp fracture line was
seen at day 1, after fracture. At day 7, fracture line became
invisible due to early intramembranous ossification.
As shown in radiographic images, callus formation was
observed at day 14 which became denser at day 21 due
to remodeling of cartilaginous callus or endochondral ossification. However, bone fracture appeared not united
even after 21 days (Figure 1B). |
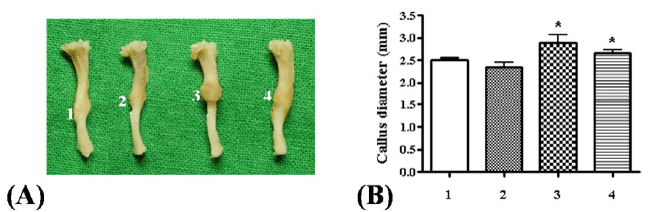 |
Figure 2. Effect of different dose of Kaempferol on callus formation in mice tibia fractures (A-B) Representative picture of tibia with external morphology of callus and callus diameter measurement in each group (1: control group; 2: administration of Kaempferol at 0.2 mg/kg; 3: Administration of Kaempferol at 0.5 mg/kg; 4: Administration of Kaempferol at 5 mg/kg) (*p<0.05) |
Effect of Kaempferol Treatment on Callus Diameter |
Mice were divided into groups as described in materials
and methods. At day 21, mice were sacrificed and
dissected. The tibiae, which had callus formation, were
collected from the hind legs. The representative picture
of tibia from each group was taken and an obvious
callus formation around the fracture site was detected
(Figure 2A). As shown in Figure 2B, significant increase
in callus diameter in groups treated with 0.5 and 5 mg/
kg of Kaempferol was observed as compared to control
group. Specially, the group with 0.5 mg/kg of Kaempferol
treatment revealed maximal callus diameter, a significant
increment of 15% compared to control group. |
Effect of Kaempferol Treatment on Callus Histology |
We next examined the callus histology of tibiae by
H&E staining (materials and methods) for endochondral
ossification and remodeling phase of bone repair at
fracture site. Representative histological sections of the
fracture sites on day 21 post-fracture are presented in
Figure 3. Histomorphological data displayed that calluses
were composed primarily of bone, implicating that most
of cartilage matrix was replaced by woven bone after 21
days of fracture induction. It was observed that there is
more significant new bone formation in samples from
Kaempferol treated groups compared to control group.
Dose of 0.5 mg/kg of Kaempferol induced maximal
new bone formation (the orange color indicates new
bone formation). Histomorphology of callus from 0.5
mg/kg Kaempferol treated group revealed that new
bone formation occurs due to enhanced endochondral
ossification and initiation of bone remodeling phase with
activities of bone cells (nucleus of osteoblasts, osteoclasts
and osteocytes as shown by the purple color) (Figure 3B). |
Effect of Kaempferol Treatment on Callus Morphology |
Treatment of 0.5 mg/kg of Kaempferol generated maximal
callus diameter and largest area of new bone formation.
To determine whether an increase in callus diameter and
new bone formation are associated with early period of
healing process of fracture, the group treated with 0.5 mg/kg of Kaempferol was examined at early time points (11
and 14 days) with respect to control group. Radiographs
(Figures 4A and 4B), taken at two time points indicate that
calluses from the treated group appeared both larger and
denser than calluses from control group. Radiographs of
tibiae in control group showed no visible callus formation
at 11 days of fracture healing; however in the treated
group early callus formation was recorded. After 14
days, calluses from the group with Kaempferol treatment
appeared denser and bigger in size than that from control
group. Taken together, data indicates that treatment with
Kaempferol can induce enhanced early healing of the
fractured site compared to control. |
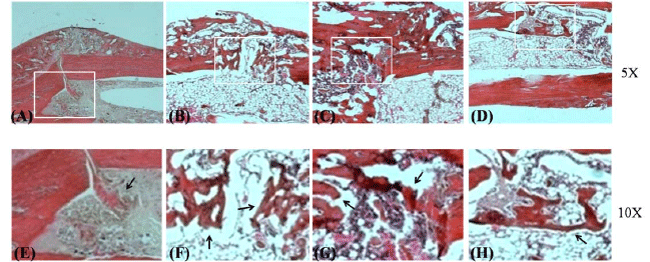 |
Figure 3. Comparison of histology of callus in different mice groups by H&E staining
(A,E) control group; (B,F) administration of Kaempferol at 0.2 mg/kg; (C,G) administration of Kaempferol at 0.5 mg/kg; (D,H)
administration of Kaempferol at 5 mg/kg. Arrows indicate new bone formation at fracture sites. |
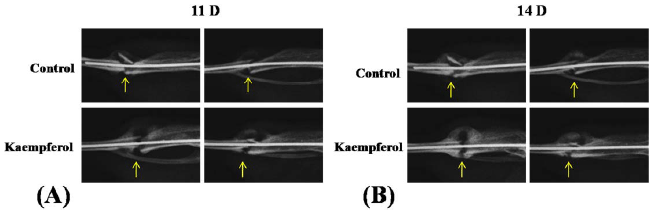 |
Figure 4. Comparison of radiography imaging between Kaempferol treated group and control group during fracture healing.
(A-B) Radiographs were taken at 11th day (11D) and 14th day (14D) post- fracture. Arrows indicate fracture sites |
Discussion |
Bone fracture is a major health issue faced by people
of almost all countries. Recovery procedure, known
as fracture healing, is a complex process driven by
many factors such as cell activities, cytokines, growth
factors etc. and is responsible for bone union at fracture
site. Occasionally, delayed union or nonunion of bone
occurs due to impaired fracture healing. Rates of bone
nonunion vary with an average of 5 to 10% in different
types of fractures and tibia shaft fracture is a common
fracture with high rate of nonunion up to 18.5% [30]. For
managing fracture healing, clinically there are two major
strategies. First is the operative method which utilizes
bone grafts as implants for fixation and augment bone
healing. Second strategy is to utilize therapeutic agents to enhance rate and quality of bone healing process. In the
aspect of therapeutic drugs, there is no registered agent to
deal with fracture healing, till date. Currently, researchers
are trying to find potential drug candidates for stimulating
bone healing from natural sources due to the safety and
economic benefits associated with them. Numerous
bioactive compounds have been reported to have positive
effects on osteogenesis, osteoblast function and bone
health such as phytoestrogen; Genistein, Quercetin,
Kaempferol and Naringin [19, 31, 32, 33]. Therefore,
taking into consideration of the advantages and positive
effect possessed by natural molecules on bone healing,
we have tried to observe the effect of natural flavonol,
Kaempferol on fracture healing process. |
Kaempferol is a promising flavonoid that has been
studied for its effect on osteogenesis and bone formation.
Kaempferol induces osteogenesis in human primary
mesenchymal stem cells as well in rat primary osteoblasts
and bone formation in newborn rat calvaria [23, 25, 26].
Moreover, Kaempferol has been shown to stimulate
chondrogenic differentiation process and cartilage
formation [27]. For the first time, our study demonstrates
the positive effect of Kaempferol on fracture healing.
The effect of Kaempferol was examined by using a
standardized mice fracture model. Kaempferol treatment
to mice with fractured tibia showed increased callus
size as well as stimulated callus formation during early
healing process of fracture. Tibiae collected after 21 days from two groups either treated with 0.5 mg/kg or 5 mg/
kg of Kaempferol had callus diameter significant larger
than that from control group. Specifically, group with 0.5
mg/kg of Kaempferol treatment had maximal diameter of
callus with an increment of 15% compared with control.
The other evidence for enhanced bone healing comes
from histomorphological analysis where callus at 21 days
post-facture showed an increase in new bone formation
in all treated groups compared with control. Group with
Kaempferol treatment of 0.5 mg/kg demonstrated maximal
bone formation. From accumulating studies including
ours, it may be hypothesized that Kaempferol has the
ability to induce callus formation and callus remodeling
during fracture healing. This effect may at least involve
increase in osteogenic activity of mesenchymal stem cells
and osteoblasts to form hard callus or may potentiate
chondrogenic activity to form soft callus. In other words,
recruitment of mesenchymal stem cells and initiation of
osteogenic process at fracture site may be stimulated by
Kaempferol. Nevertheless, it needs to be verified by more
detailed studies elaborating on the signaling mechanism
involved for its osteogenic activity. In addition to the
effectiveness in promoting bone healing, efficacy and
pharmacokinetics of these compounds at clinical level
also needs to be elucidated. |
Radiographical imaging of calluses from 0.5 mg/kg of
Kaempferol treated group appeared both larger and denser
than calluses from control group at 11 days and 14 days
post-fracture. Callus formation and remodeling of callus
are important processes for recovering a fractured site.
Our data displayed that Kaempferol at an optimum dose
of 0.5 mg/kg affects the callus formation both in term
of size as well the initial time needed for healing, and
stimulates remodeling of callus by promoting conversion
of cartilaginous callus to woven bone. Data obtained here
clearly implicates that Kaempferol has a potential to initiate
and enhance fracture healing in mice tibia, though, further
researches focused on the biomechanical properties of the
healed area may be more supportive and require elaborate
experimental set up. A view about the microarchitecture
and biomechanical properties of fractured site is desirable
to predict the bone strength which can help to assess the
abilities to perform routine physical activities. |
Flavonoids are known to have many good effects on bone
health [34, 35]. Recently, a study of Huh et al., suggested
that formononectin promotes femur fracture healing in
rat by increasing angiogenesis and osteogenesis [16].
Similarly, Kaempferol may improve fracture healing by
enhancing callus remodeling process for increasing new
bone formation. While, phytoestrogens and formononectin
have been shown to possess estrogenic effects [36, 37],
Kaempferol appears to be more safe as it is reported to
possess no estrogenicity at uterine level [17], ruling out
any kind of adverse effect due to estrogenic properties. Taken together, our studies suggest that Kaempferol is
a potential therapeutic agent for fracture healing with
potential safety and effectiveness associated with it. |
Conclusion |
Fracture healing process often suffers from nonunion or
delayed union due to various clinical abnormalities like
osteoporosis. Till date, no effective drugs are available
for promoting bone healing in such conditions. Recently
a number of phytoestrogens have been shown to promote
osteogenesis and bone formation in vitro and in vivo. In
here, we have assessed ability of a flavanol, Kaempferol
in potentiating the bone healing process in tibia shaft
fracture model of mice. Results obtained demonstrate that
Kaempferol enhanced the callus formation and diameter
at fracture sites in mice. Moreover, histo-morphological
analysis demonstrated recruitment of cells favoring bone
formation at fracture site after the treatment of Kaempferol.
A dose of 0.5 mg/kg was found to be most effective
compared to control. Future studies focused on elucidating
the mechanism of action as well as the biomechanical
properties of the healed fracture site by Kaempferol can
provide more supportive information about the potential
of Kaempferol for fracture healing. |
Acknowledgements |
This research was supported by Basic Science Research
Program through the National Research Foundation
of Korea (NRF) funded by the Ministry of Education
(2014R1A1A4A03009388 & 2014R1A1A2055560), by
a grant of the Korea Health Technology R&D Project
through the Korea Health Industry Development Institute
(KHIDI), funded by the Ministry of Health & Welfare,
Republic of Korea (HI12C1265), and by Hallym
University Research Fund. |
Conflict of Interest |
There is no conflict of interest. |
References |
- Schindeler A, McDonald MM, Bokko P, Little DG. Bone remodeling during fracture repair: The cellular picture. Semin Cell Dev Biol 2008;19: 459-466.
- Stuermer EK, Sehmisch S, Rack T, Wenda E, Seidlova-Wuttke D. Estrogen and raloxifene improve metaphyseal fracture healing in the early phase of osteoporosis. A new fracture-healing model at the tibia in rat. Langenbecks Arch Surg 2010;395: 163-172.
- Lyritis G, Boscainos PJ. Calcitonin effects on cartilage and fracture healing. J Musculoskelet Neuronal Interact 2001;2: 137-142.
- Kyllonen L, D'Este M, Alini M, Eglin D. Local drug delivery for enhancing fracture healing in osteoporotic bone. Acta Biomater 2015;11: 412-434.
- Kakar S, Einhorn TA, Vora S, Miara LJ, Hon G. Enhanced chondrogenesis and Wnt signaling in PTH-treated fractures. J Bone Miner Res 2007;22: 1903-1912.
- Simpson AH, Mills L, Noble B. The role of growth factors and related agents in accelerating fracture healing. J Bone Joint Surg Br 2006;88: 701-705.
- Moroni A, Faldini C, Hoang-Kim A, Pegreffi F, Giannini S. Alendronate improves screw fixation in osteoporotic bone. J Bone Joint Surg Am 2007;89: 96-101.
- Gerstenfeld LC, Sacks DJ, Pelis M, Mason ZD, Graves DT. Comparison of effects of the bisphosphonate alendronate versus the RANKL inhibitor denosumab on murine fracture healing. J Bone Miner Res 2009;24: 196-208.
- Glass GE, Jain A. Cochrane corner: bone morphogenetic protein (BMP) for fracture healing in adults. J Hand Surg Eur Vol 2013;38: 447-449.
- Bigham-Sadegh A, Oryan A. Basic concepts regarding fracture healing and the current options and future directions in managing bone fractures. Int Wound J 2015;12: 238-247.
- Brandi ML. Drugs for bone healing. Expert Opin Investig Drugs 2012;21: 1169-1176.
- Hsueh TP, Chiu HE. Traditional Chinese medicine speeds-up humerus fracture healing: two case reports. Complement Ther Med 2012;20: 431-433.
- Mohammad S, Pal US, Pradhan R, Singh N. Herbal remedies for mandibular fracture healing. Natl J Maxillofac Surg 2014;5: 35-38.
- Chow SP, Yeung HW, Law LK, Chan TM, Lau C. The effect of Davallina Orientalis on bone healing--a preliminary report. Am J Chin Med 1982;10: 101-106.
- Ozturk A, Ilman AA, Saglam H, Yalcinkaya U, Aykut S. The effects of phytoestrogens on fracture healing: experimental research in New Zealand white rabbits. Ulus Travma Acil Cerrahi Derg 2008;14: 21-27.
- Huh JE, Kwon NH, Baek YH, Lee JD, Choi DY. Formononetin promotes early fracture healing through stimulating angiogenesis by up-regulating VEGFR-2/Flk-1 in a rat fracture model. Int Immunopharmacol 2009;9: 1357-1365.
- Trivedi R, Kumar S, Kumar A, Siddiqui JA, Swarnkar G. Kaempferol has osteogenic effect in ovariectomized adult Sprague-Dawley rats. Mol Cell Endocrinol 2008;289: 85-93.
- Miyake M, Arai N, Ushio S, Iwaki K, Ikeda M. Promoting effect of Kaempferol on the differentiation and mineralization of murine pre-osteoblastic cell line MC3T3-E1. Biosci Biotechnol Biochem 2003;67: 1199-1205.
- Prouillet C, Maziere JC, Maziere C, Wattel A, Brazier M. Stimulatory effect of naturally occurring flavonols quercetin and Kaempferol on alkaline phosphatase activity in MG-63 human osteoblasts through ERK and estrogen receptor pathway. Biochem Pharmacol 2004; 67: 1307-1313.
- Kumar A, Gupta GK, Khedgikar V, Gautam J, Kushwaha P. In vivo efficacy studies of layer-by-layer nano-matrix bearing Kaempferol for the conditions of osteoporosis: a study in ovariectomized rat model. Eur J Pharm Biopharm 2012;82: 508-517.
- Lee WS, Lee EG, Sung MS, Yoo WH. Kaempferol inhibits IL-1beta-stimulated, RANKL-mediated osteoclastogenesis via downregulation of MAPKs, c-Fos, and NFATc1. Inflammation 2014;37: 1221-1230.
- Wattel A, Kamel S, Mentaverri R, Lorget F, Prouillet C. Potent inhibitory effect of naturally occurring flavonoids quercetin and Kaempferol on in vitro osteoclastic bone resorption. Biochem Pharmacol 2003;65: 35-42.
- Yang L, Takai H, Utsunomiya T, Li X, Li Z. Kaempferol stimulates bone sialoprotein gene transcription and new bone formation. J Cell Biochem 2010;110: 1342-1355.
- Suh KS, Choi EM, Kwon M, Chon S, Oh S. Kaempferol attenuates 2-deoxy-d-ribose-induced oxidative cell damage in MC3T3-E1 osteoblastic cells. Biol Pharm Bull 2009;32: 746-749.
- Guo AJ, Choi RC, Zheng KY, Chen VP, Dong TT. Kaempferol as a flavonoid induces osteoblastic differentiation via estrogen receptor signaling. Chin Med 2012;7: 10.
- Byun MR, Jeong H, Bae SJ, Kim AR, Hwang ES. TAZ is required for the osteogenic and anti-adipogenic activities of Kaempferol. Bone 2012;50: 364-372.
- Nepal M, Li L, Cho HK, Park JK, Soh Y. Kaempferol induces chondrogenesis in ATDC5 cells through activation of ERK/BMP-2 signaling pathway. Food Chem Toxicol 2013;62: 238-245.
- Hiltunen A, Vuorio E, Aro HT.A standardized experimental fracture in the mouse tibia. J Orthop Res 1993;11: 305-312.
- Carmouche JJ, Puzas JE, Zhang X, Tiyapatanaputi P, Cory-Slechta DA, Gelein R, Zuscik M, Rosier RN, Boyce BF, O'Keefe RJ. Lead exposure inhibits fracture healing and is associated with increased chondrogenesis, delay in cartilage mineralization, and a decrease in osteoprogenitor frequency. Environ Health Perspect 2005;113: 749-755.
- Fong K, Truong V, Foote CJ, Petrisor B, Williams D. Predictors of nonunion and reoperation in patients with fractures of the tibia: an observational study. BMC Musculoskelet Disord 2013;14: 103.
- Trivedi R, Kumar A, Gupta V, Kumar S, Nagar GK. Effects of Egb 761 on bone mineral density, bone microstructure, and osteoblast function: Possible roles of quercetin and Kaempferol. Mol Cell Endocrinol 2009;302: 86-91.
- Heim M, Frank O, Kampmann G, Sochocky N, Pennimpede T. The phytoestrogen genistein enhances osteogenesis and represses adipogenic differentiation of human primary bone marrow stromal cells. Endocrinology 2004;145: 848-859.
- Wong RW, Rabie AB. Effect of naringin on bone cells. J Orthop Res 2006;24: 2045-2050.
- Taku K, Melby MK, Takebayashi J, Mizuno S, Ishimi Y. Effect of soy isoflavone extract supplements on bone mineral density in menopausal women: meta-analysis of randomized controlled trials. Asia Pac J Clin Nutr 2010;19: 33-42.
- Welch AA, Hardcastle AC. The effects of flavonoids on bone. Curr Osteoporos Rep 2014;12: 205-210.
- Santell RC, Chang YC, Nair MG, Helferich WG. Dietary genistein exerts estrogenic effects upon the uterus, mammary gland and the hypothalamic/pituitary axis in rats. J Nutr 1997;127: 263-269.
- Mu H, Bai YH, Wang ST, Zhu ZM, Zhang YW. Research on antioxidant effects and estrogenic effect of formononetin from Trifolium pratense (red clover). Phytomedicine 2009;16: 314-319.
|