Keywords |
Heart-Hypothyroidism, Thymoquinone-Nitric oxide, Reactive oxygen species, Histopathology |
Introduction |
Nigella sativa (NS), the promising medicinal plant,
commonly known as black seed, was medicinally used for
over 2000 years in many middle eastern and eastern Asian
countries [1]. NS seeds or its oil was used in the treatment
of many diseases, including asthma, inflammations,
hypertension and diabetes [2]. Thymoquinone (TQ), the
biologically active substance of NS seeds and its oil, was
found to possess powerful antioxidant and antiinflammatory
activities [3,4]. |
Thyroid hormones were considered one of the important modulators of the cardiovascular function. In addition,
the heart was the target organ of the thyroid hormones
[5]. Hypothyroidism, was a syndrome caused by thyroid
hormone deficiency. Recently, Ohga et al. [6], reported
left ventricular systolic and diastolic dysfunction in
propylthiouracil (PTU)-induced hypothyroidism in rats.
Recently, Knapp et al. [7] indicated that hypothyroidism
resulted in oxidative stress, and heart diseases. In
particular, it was suggested that, hypothyroidism
increased the formation of reactive oxygen species
(ROS) in the heart and consequently, increased lipid
peroxidation [8]. |
In this study, it is hypothesized that hypothyroidisminduced
cardiac pathological changes are caused via
oxidative stress mechanism and hence, the use of an
antioxidant may protect the heart against these changes.
This study aimed to reassess, hypothyroidism-induced
cardiac histopathological changes and to evaluate the
proposed protective role of thymoquinone (TQ) against
these changes. In addition, the mechanism of TQ-induced
protection is studied regarding the oxidative stress and
nitric oxide (NO) pathway. |
Materials and Methods |
Chemicals |
Thymoquinone from NS (28 mg) was obtained from
FRINTON LABORATORIES, INC. It is a yellow
powder, which was dissolved in DMSO and diluted
to 1:100 in a sterile saline solution (0.9% NaCl) to
produce a working stock. PTU was purchased from
Sigma Aldrich lnc, USA. |
Animals and treatments |
This study was approved by the Biomedical Ethics
Research Committee at the Faculty of Medicine, King
Abdulaziz University, Jeddah, Saudi Arabia. Adult male
Wister rats (180-200 g) were obtained from the Animal
Resources Division of King Fahd Medical Research Center.
The rats were housed at 22 ± 3°C and relative humidity of
44%-55% with a 12 h dark/light cycle and were provided
with standard laboratory feed and water ad libitum.
The animals received care, according to institutional
guidelines for the care and use of the laboratory animals in
King Fahd Medical Research Center. Rats were randomly
divided into 4 groups (n=6 each). The control group: rats
received normal saline (6 weeks, 2 ml/kg/day, per oral;
po); TQ group: rats received TQ (6 weeks, 10 mg/kg/day
po) [9]; PTU (hypothyroidism) group: rats received (6
weeks, 6 mg/kg/day, po) as was previously described [10]
and PTU+TQ group: received PTU (6 weeks, the same
dose, po) and TQ (10 mg/kg/day, po) [11] starting from the
2nd week to 6th week. Body weight of the rats was assessed
at the start and at the end of the experiment. |
Sample collection |
At the end of the 6th week, the rats were decapitated under
light ether anesthesia, blood samples were withdrawn by
heart puncture. Blood samples were centrifuged and the
serum were kept at -80°C until used for the biochemical
analysis. Hearts were dissected out, weighted and
processed for histopathological examination. |
Measurement of serum triiodothyronine (T3), thyroxine
(T4) and thyroid-stimulating hormone (TSH) |
Serum T3, T4 and TSH concentrations were measured in
El-Safwa Laboratory; Tanta, Egypt, using ADVIA Centaur
automated competitive chemiluminescence immunoassay
(Bayer HealthCare). |
Measurement of plasma lactate dehydrogenase (LDH)
and creatine phosphokinase CPK) |
Total plasma LDH and CPK activities were measured using
kits of Biodiagnostic, Egypt. LDH activity was measured
according to Pesce [12] and the method of Abbot et al.
[13] was adopted to determine the CPK enzyme activity. |
Measurement of plasma lipid peroxide (measured as
malondialdehyde (MDA), reduced glutathione (GSH),
nitric oxide (NO), glutathione peroxidase enzyme activity
(GPx), superoxide dismutase enzyme activity (SOD) and
catalase enzyme activity (CAT) |
Plasma MDA was measured using Biodiagnostic kits,
Egypt, according to Uchiyama and Mihara [14]. Plasma
GSH was quantified using Biodiagnostic kits, Egypt,
according to Ellman [15]. Plasma NO was measured using
Biodiagnostic kits, Egypt, according to Tarpey et al. [16].
Plasma GPx activity was measured using Biodiagnostic
kits, Egypt, according to Paglia and Valentine [17]. Plasma
SOD activity was measured using Biodiagnostic kits,
Egypt, according to Nishikimi et al. [18]. Plasma CAT
activity was measured using Biodiagnostic kits, Egypt,
according to Aebi [19]. |
Histopathological examination of the heart |
After processing the heart for histopathological
examination, paraffin blocks were obtained and serially
sectioned (3-5 µm), then stained with hematoxylin and
eosin (H and E) and Masson?s trichrome (MT) stains
[20]. The cross sectional area of the cardiomyocytes in
the left ventricle was measured in 30 field per animal at
magnification 100X objective lens and 10X ocular lens
using Pro Plus image analysis software version 6.0. The
area percent of the MT-stained collagen fiber (stained blue
in color) was assessed using the same software as was
described by Afifi and Hanon [21]. The histopathologist
who examined the slides was blind to the groups studied. |
Immunohistochemical examination of heart sections |
Immunohistochemical staining was done using
streptavidine?biotin?peroxidase technique according to
Bancroft [20]. Alpha smooth muscle actin (ASMA; Dako
Cytomation, Heverlee, Belgium, at a dilution 1/1000),
desmin (Dako, Trappes, Frances, at a dilution 1/100)
and endothelial nitric oxide synthetase (eNOS; Abcam,
Cambridge, MA at a dilution 1/50) antibodies were
used in this study. The nuclei were counterstained with
hematoxylin. Semiquantitative analysis of the extension
of the immunoreactivity was determined by assessing the
area percentage using Pro Plus image analysis software
version 6.0. ASMA, desmin, and NOS area percent was
assessed in 30 field per animal at magnification 40X
objective lens and 10X ocular lens as described by Leslie
et al. [22]. On assessing the area percent of ASMA or MTstained
collagen fibers, fields with blood vessels were
excluded from assessment. |
Statistical Analysis |
SPSS program (Version 16) was used to statistically
analyze all data. The results were expressed as mean ±
SDM. Comparisons between different groups were carried
out by one-way analysis of variance (ANOVA) followed
by Tukey-Kramer test for the parametric data. The
morphometric and image analysis data (non-parametric)
were analyzed by Kruskal-Wallis ANOVA followed
Dunn's test. Person correlation was made between the non-
parametric variable. Statistical significance was accepted
at p = 0.05. |
Results |
The effect of PTU and TQ on body and heart weights,
serum total T3, T4 and TSH concentrations |
Adminstration of PTU significantly reduced (p=0.03) the
rats weight gain while it significantly increased (p=0.02)
the relative weight of the heart compared to the control.
Adminstration of TQ to PTU-treated rats resulted in
significant decrease (p=0.04) in the relative weight of the
heart compared to the PTU group (Table 1). |
Treatments of rats with PTU caused a significant decrease
in both serum T3 and T4 concentrations (15% and 51%,
respectively) compared to the control concentrations
(p=0.000 and 0.008, respectively) (Table 1). PTU resulted
in a significant increase in serum TSH concentration (~
6 fold) compared to the control concentration (p=0.001)
(Table 1). Adminstration of TQ to PTU-treated rats
resulted in a significant increase in both serum T3 and
T4 concentrations (21% and 82%, respectively) and a
significant decrease in serum TSH concentration (87%) compared to PTU injected rats (p=0.01; 0.004 and 0.001
respectively) (Table 1). |
Effect of TQ on plasma LDH and CPK activities in PTU-
induced hypothyroidism |
Treatments of rats with PTU significantly increased
both plasma LDH and CPK activities (64% and 73%,
respectively) compared to the control activities (p=0.000
and 0.009, respectively) (Table 2). TQ significantly
decreased both plasma LDH and CPK activities (42% and
75%, respectively) in PTU=TQ group compared to PTU-
treated rats (p=0.045 and 0.008, respectively) (Table 2). |
Effect of TQ on plasma MDA, GSH and NO
concentrations in PTU-induced hypothyroidism |
Treatments of rats with PTU significantly increased both
plasma MDA and NO levels (~ 3 and 2 fold, respectively)
compared to the control levels (p<0.001 and 0.02,
respectively) (Figure 1). On the other hand, treatments of
rats with PTU caused a nonsignificant decrease (25%) in
plasma GSH concentrations compared to the control rats
(p=0.535) (Figure 1). Treatment with TQ after 2 weeks
of PTU administration caused a significant decrease
(p=0.027) in plasma MDA concentrations (31%) and a
significant increase in plasma NO and GSH concentrations
(22% and 77%, respectively) compared to PTU treated
rats (p=0.016 and 0.035, respectively) (Figure 1). |
Effect of TQ on plasma GPx, SOD and CAT activities in
PTU-induced hypothyroidism |
The results of enzymatic antioxidant analyses were
shown in (Table 3). Briefly, the activities of GPX were
not changed in all treatment regimens. Treatments of rats with PTU significantly decreased plasma SOD activity
(63%) compared to the control rats (p=0.000). Treatment
with TQ after 2 weeks of PTU adminstration significantly
increased plasma SOD activity (~ 2 fold) compared to
PTU injected rats (p=0.035). In addition, treatments of
rats with PTU caused significantly increased plasma CAT activity (84%) compared to the control rats (p=0.000).
Treatment with TQ after 2 weeks of PTU administration
caused a nonsignificant decrease in plasma CAT activity
(6%) compared to PTU injected rats (p=0.599). |
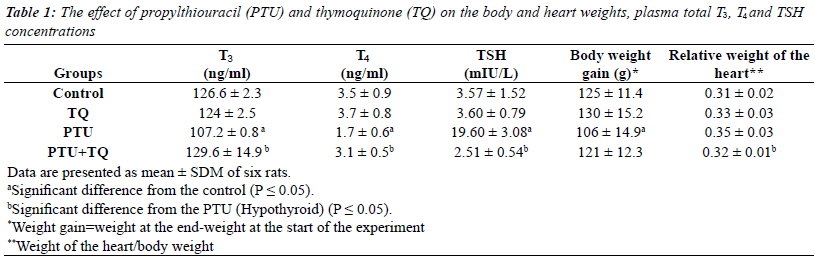 |
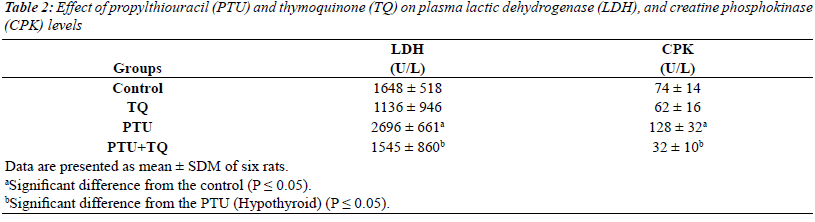 |
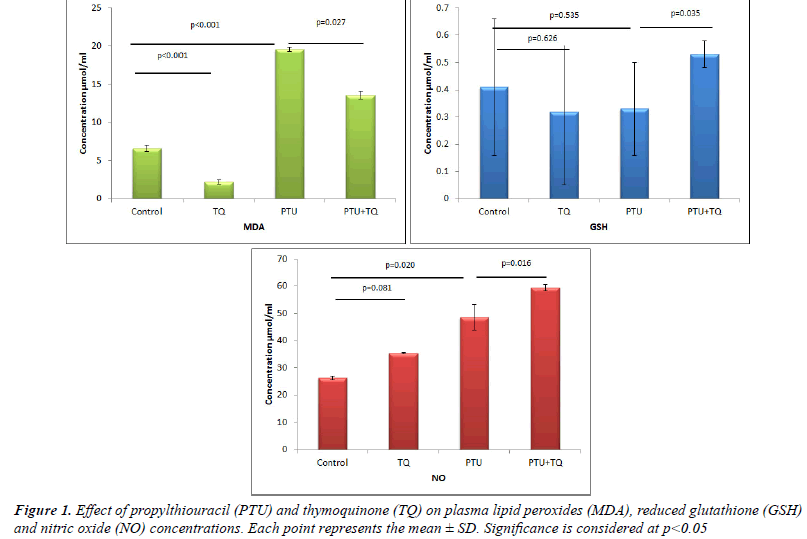 |
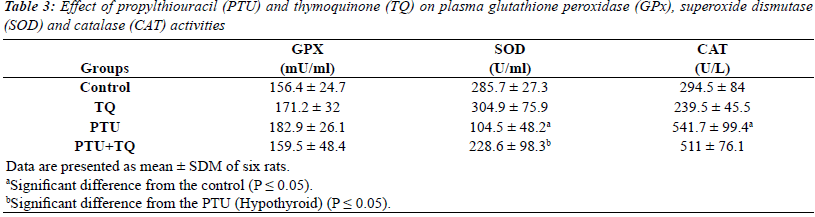 |
Effect of TQ on histopathological changes of hearts in
PTU-induced hypothyroidism |
Left ventricle of the control rats showed intact branching
and anastomosing cardiac muscle fibers (CMFs) which
possess acidophilic sarcoplasm with regular striations and
oval central nuclei. Left ventricular CMFs of TQ group
showed no histological changes compared with that of the control group Figure (2A, B). The left ventricle of PTU
group showed some myocytes with hydropic changes in
the form of lost striations and pyknotic nuclei. Continuity
of the adjacent myocytesas well as lateral alignment of the
myofibrils was lost in some areas together with nuclear
displacement. Cellular infiltration and hemorrhage were
also observed. The cross sectional area of the CMFs of
this group was significantly decreased compared with that
of the control rats (Figure 2C-E, G). The PTU+TQ group
showed intact myocytes in almost all left ventricle with
fewer cellular infiltrate and hemorrhages compared to the
PTU group and the cross sectional area of the CMFs was
insignificantly increased compared with that of the PTU
group (Figure 2F). A significant increase in area percent of
collagen fibers was observed in the left ventricle of PTU
group compared with that of the control group while the
PTU+TQ group showed a significant decrease compared
with that of PTU group (Figure 3). In addition, significant positive correlations existed between area percent of
collagen fibers and blood levels of LDH (r=0.532, p=0.01)
and CPK (r=0.496, p=0.01). |
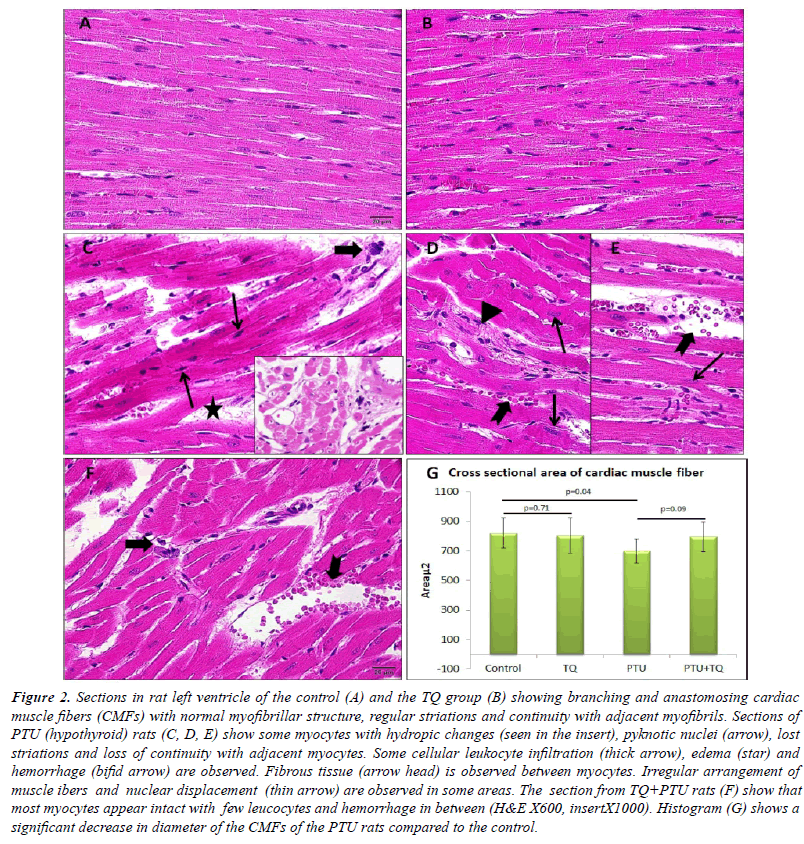 |
Effect of TQ on PTU-induced immunohistochemical
changes in ASMA, desmin and eNOS expression |
Blood vessel wall showed strong ASMA expression
in the left ventricle of both the control and TQ groups,
whereas the CMFs showed negative expression. Some
CMFs of PTU group showed moderate ASMA expression
while fewer CMFs showed weak expression in the
PTU+TQ group. Both groups showed many branched
myofibroblasts between the CMFs with a strong ASMA expression. A significant increase (p<0.001) was observed
in ASMA expression in PTU group compared with the control group and TQ administration significantly reduced
it (p<0.001) (Figure 4). A significant positive correlation
(r=0.916, p<0.001) was recorded between area percent of
ASMA expression and that of collagen fibers in the left
ventricle (Figure 3F). In addition, significant positive
correlations existed between area percent of ASMA expression and blood levels of LDH (r=0.580, p<0.003)
and CPK (r=0.678, p<0.001). |
A strong desmin expression was observed in CMFs of
both control and TQ groups. Most of CMFs of PTU and
PTU+TQ groups showed a strong desmin expression while
few of them showed weak or no expression. A significant
decrease (p<0.001) in desmin expression was observed in the left ventricular CMFs of PTU group compared with
the control, whereas those in the PTU+TQ group showed
a significant increase (p<0.05) compared with the PTU
group (Figure 5). |
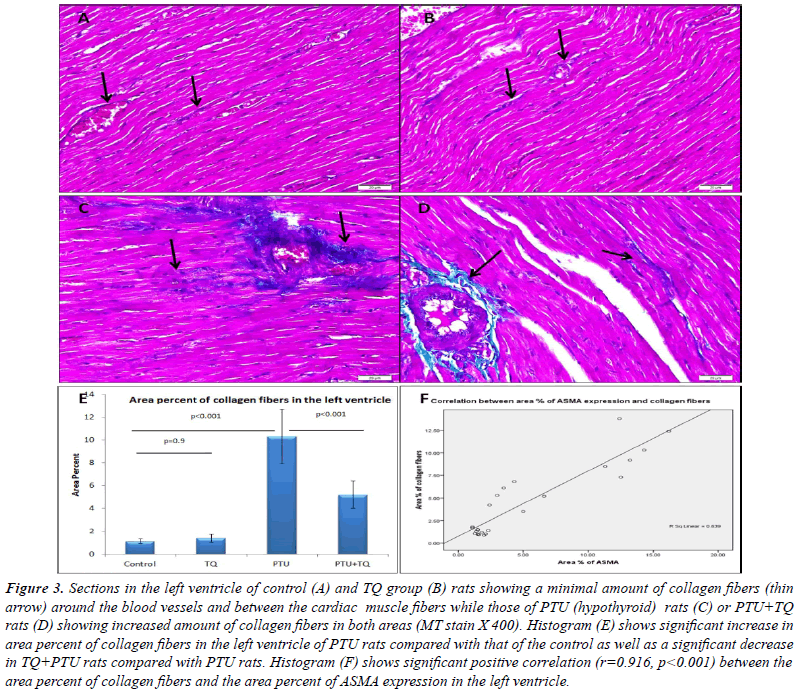 |
Concerning eNOS, a moderate expression was observed in
the CMFs and the endothelial lining of the blood vessels
of the control group. PTU group showed an increased
expression that was found to be statistically significant
(p=0.04) when compared to the control group. The left
ventricle of the PTU+TQ group showed strong eNOS
expression that was significantly higher (p=0.01) when
compared to those of the PTU group (Figure 5). |
Discussion |
This study was conducted first to reassess the impact of
experimentally-induced hypothyroidism on the adult
rat heart. An experimental model of thyroid hormone
deficiency was made by the reversible goitrogen PTU which
decreased the conversion of T4 to T3. The hypothyroid
state was ensured as the serum T3 and T4 levels were
significantly lower, whereas the serum TSH level was
significantly higher compared to control rats. A significant increase in plasma LDH and CPK enzymes activity was
recorded in hypothyroid rats compared to control rats
which indicate cardiac muscle affection. This was found to
be accompanied by significant histopathological changes in
left ventricle included hydropic changes in CMFs, cellular
infiltration and multiple hemorrhages. These findings
were in line with those of Massoud et al. [23]. In this
study the hypothyroid rats weight gain was significantly
reduced and this was in line with the previous studies
[24]. On the other hand, the relative weight of the heart
was significantly increased and this could be attributed to
the infiltration of the inflammatory cell observed on the
microscopic examination. |
In the present study, a significant decrease in the CMFs cross sectional area was observed. In previous studies myocardial
atrophy in hypothyroidism and marked reduction in muscle
fiber mass in the PTU (hypothyroid) rats myocardium was reported [22]. This myocardial atrophy could be explained in the light of Klein and Danzi findings [25]. They reported that thyroid hormone increased total protein synthesis in
the cardiac muscle and controls the transcription myosin
heavy chain genes that are important for heart function. |
A significant increase in ASMA expression was observed in the left ventricle of hypothyroid rats in this study
indicating further fibrosis. The area percent of collagen fibers was positively correlated with ASMA expression in all the studied groups, suggesting that the collagen fibers were derived from cells expressing ASMA. This was in line with a recent study which reported that in myocardial
stress, and heart diseases, cardiomyocytes are lost due to the myofibroblasts initiated reparative fibrosis with subsequent increase in ASMA-positive cells [26]. It was described that ASMA, a protein that is present in embryonal/fetal heart muscle cells, but absent in adult cardiomyocytes, is
reexpressed in cardiomyocytes, undergo dedifferentiation, during heart hypertrophy induced by cardiac overload [27] and this could explain for ASMA expression observed in some left ventricular myocytes in this study. |
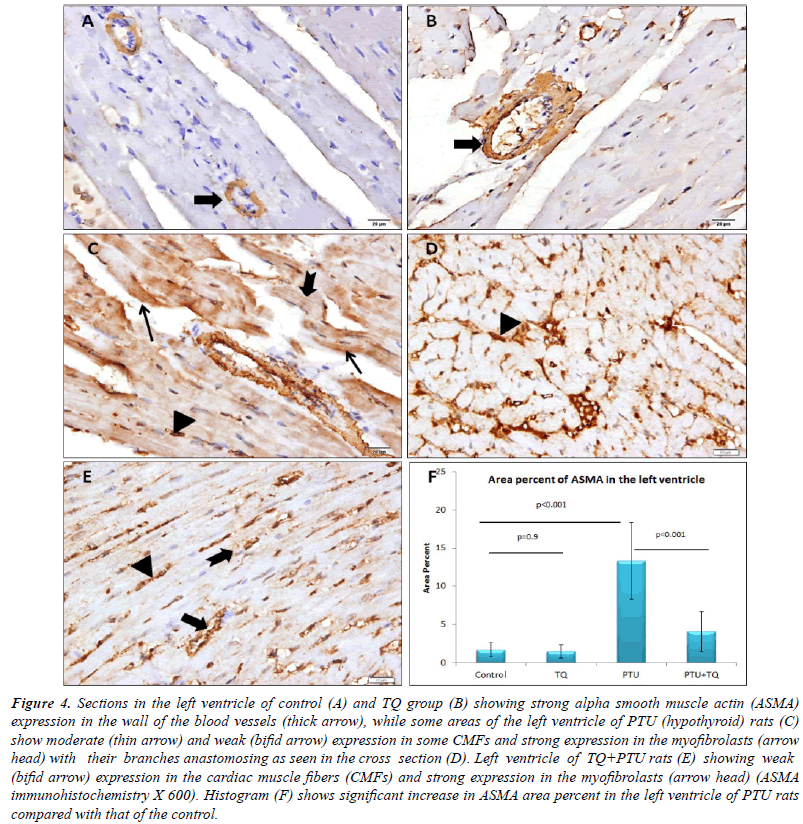 |
The significant decrease in desmin expression was
observed in the left ventricle CMFs of hypothyroid
rats, in this study, indicating affection of the structural
integrity of these fibers as was reported by Paulin et al.
[28]. In addition, Capetanaki et al. [29] reported that mice
lacking desmin develop numerous muscle architectural
and ultrastructural defects, especially in extensively
used muscles such as the heart and among the structural
abnormalities observed were loss of lateral alignment of
myofibrils and loss of nuclear shape and positioning that
was observed in the present study. |
The second aim of the present work was to assess
the suggested cardioprotective action of TQ against
hypothyroidism-induced cardiac affection. In this study,
treatment of rats with TQ significantly protects the heart muscle against hypothyroidism-induced histopathological
and immunohistochemical changes. It also significantly
decreased plasma LDH and CPK enzymes activity
compared to PTU group. This study reported a protective
effect of TQ against PTU-induced hypothyroidism and
the associated cardiac damage and this is supported by
Nagi and Mansour [30] who reported a protective role of
thymoqinone against adriamycin-induced cardiotoxicity. |
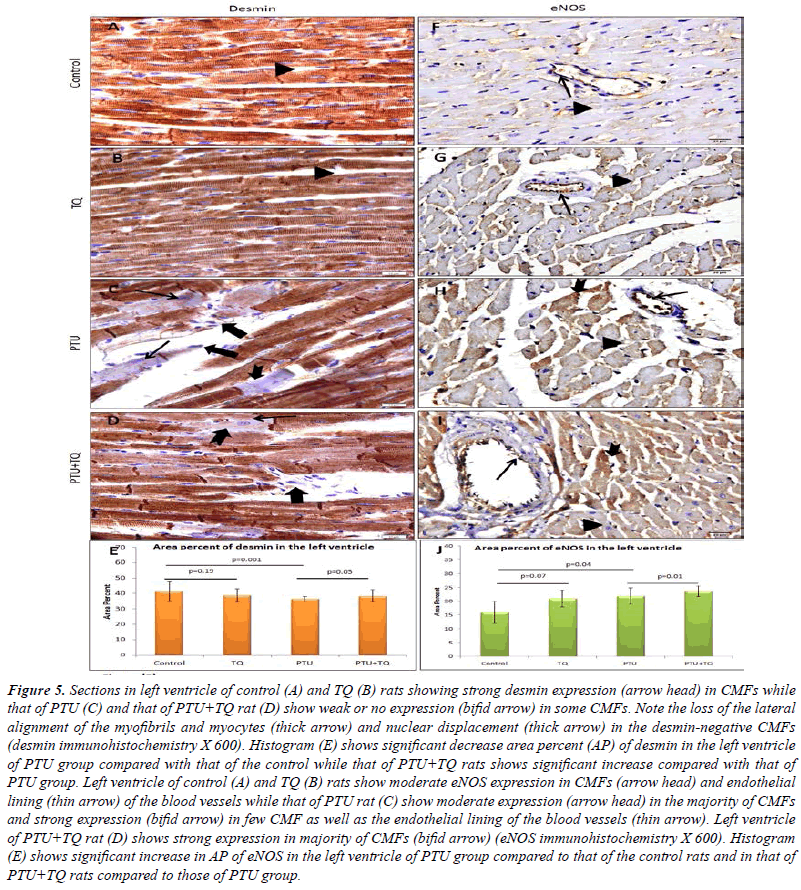 |
Finally, the mechanism of TQ -induced protection is
studied regarding the ROS and NO formation. In the
present study, the effect of PTU on T3, T4 and TSH seems
to be reversed in rats treated with TQ as the levels of the T3, T4 and TSH tends to be near the normal levels. This finding
is in line with that of Shariatifar et al. [31] who reported
that NS significantly increases the levels of T3 and T4 and
decreases the TSH in mice. The role of thyroid hormones
in metabolic pathways and antioxidant enzyme activities
was well known in many species [32]. In the present study
PTU-induced hypothyroidism caused an increase in the
plasma MDA formation (a product of lipid peroxidation)
and CAT activity and a decrease in plasma SOD activity
while both GSH content and GPX activity were not altered.
Increased MDA formation suggested enhancement of
oxidative stress in hypothyroidism. This is in line with the results of Yilmaz et al. [8], who reported increased
plasma, liver, heart and muscle MDA level in hypothyroid
rats. In addition, Chattopadhyay et al. [33] reported
increased CAT activity in hypothyroidism, heart, which
is suggested to be a reflex mechanism against increased
oxidative stress-induced by hypothyroidism. Choudhury
et al. [34] reported a fall in SOD and CAT activity in
hypothyroid rats, but in addition of T3, only the catalase
activity was restored. Chattopadhyay et al. [33] described
that, a decreased thyroid function may disturb SOD/CAT
ratio and hence imbalance the oxidant/antioxidant state
resulting in generation of oxidative stress. Petrulea et al.
[35] also reported that GSH didn't differ significantly in
serum and different tissues of hypothyroid rats and this was
observed also in this study. Thymoquinone effect seems to
be mediated through a reduction in MDA formation, and
increased GSH and SOD production, hence it restores the
oxidant/antioxidant balance. The mechanism underlying
TQ protective effect in this study could be attributed to the
normalization of T3, T4 and TSH. Thymoquinone acts as a
potent free radical scavenger against superoxide, hydroxyl
and singlet oxygen radical [36]. |
NO is an important vasodilator produced by coronary
endothelial cells, which exerts a cardioprotective effect. It
serves as an oxygen free radical scavenger, hence minimizing
the deleterious effects of the oxidative stress [37]. In
addition, it inhibits contractile tone and the proliferation
of underlying vascular smooth muscle cells and promotes
diastolic relaxation [38]. This study hypothesized a role
of NO in TQ induced protection against hypothyroidism
induced pathological cardiac changes. The present results
showed that PTU-induced hypothyroidism increased NO
formation, which seems to be a protective mechanism,
and TQ treatment resulted in a further increase in NO
formation. The immunohistochemical results were in line
with this as the eNOS expression was increased in the left
ventricle of rats received PTU and further increased in TQ
rats. This finding is in concordance with some previous
studies which reported an increase in NOS activity in both
ventricles of the chronic hypothyroidism models and in
the atria, endothelial and smooth muscle of the aorta of
the acute model of thyroidectomy [39]. Increased NOS
activity was described to be a compensatory mechanism
stimulated by decreased plasma T3 levels which plays
a protective role in maintaining blood ?ow and reduces
cardiac after load that resulted in an increase in the cardiac
output and attenuation of pulmonary edema, thus markedly
improving survival [39]. |
Conclusion |
This study concluded that hypothyroidism may induce
pathological cardiac changes, which were prevented by
TQ via increased thyroid hormones and NO, and decreased
ROS production. |
Conflict of Interest |
The authors declare that they have no conflict of interest. |
References |
- Haq A, Abdullatif M, Lobo PI, Khabar KS, Sheth KV, al-Sedairy ST. Nigella sativa: effect on human lymphocytes and polymorphonuclear leukocyte phagocytic activity.Immunopharmacolog1995; 30: 147-155.
- Ali BH, Blunden G. Pharmacological and toxicological properties of Nigella sativa. Phytother Res 2003; 17: 299-305.
- Houghton P, Zarka R, Las Heras B, Hoult J. Fixed oil of Nigella sativa and derived thymoquinone inhibit eicosanoid generation in leukocytes membrane lipid peroxidation. Plant Med 1995; 61:33-36.
- Leong XF, Rais Mustafa M, JaarinK. Nigella sativa and Its Protective Role in Oxidative Stress and Hypertension. Evid Based Complement Alternat Med 2013.
- Dillmann WH. Cellular action of thyroid hormone on the heart. Thyroid 2002; 12: 447-452.
- OhgaY, Sakata S, TakenakaC, Abe T, Tsuji T, Taniguchi S, TakakiM. Cardiac dysfunction in terms of left ventricular mechanical work and energetics in hypothyroid rats. Am J Physiol Heart CircPhysiol2002; 283: H631-641.
- Knapp M, Lisowska A, Sobkowicz B, TycinskaA, SawickiR, Musial WJ. Myocardial perfusion and intima-media thickness in patients with subclinical hypothyroidism. Adv Med Sci2013; 58: 44-49.
- Yilmaz S, Ozan S, Benzer F, Canatan H. Oxidative damage and antioxidant enzyme activities in experimental hypothyroidism. Cell BiochemFunct2003; 21: 325-330.
- Badary OA, Abdel-Naim AB, Abdel-Wahab MH, Hamada FM. The influence of thymoquinone on doxorubicin-induced hyperlipidemic nephropathy in rats, Toxicol 2000; 143: 219-226.
- Villar D, Rhind SM, Dicks P, McMillen SR, Nicol F, Arthur JR. Effect of propylthiouracil-induced hypothyroidism on thyroid hormone profiles and tissue deiodinase activity in cashmere goats. Small Ruminant Research 1998; 29: 317-324.
- EbruU, BurakU, Yusuf S, ReyhanB, ArifK, Faruk TH, EminM, AydinK, AtillaII, SemsettinS, Kemal E. Cardioprotective effects of Nigella sativa oil on cyclosporine A-induced cardiotoxicity in rats. Basic ClinPharmacolToxicol2008; 103: 574-580.
- Pesce AJ. Lactate dehydrogenase: In: Methods inClinical Chemistry. The C. V. Mosby Comany, St. Louis., 1987; pp.903-906.
- Abbot B. Creatinine kinase: In: Clinical chemistry. St Louis, Toronto, Princeton: Mosby 1984; pp.1112e6.
- Uchiyama M, Mihara M. Determination of malonaldehyde precursor in tissues by thiobarbituric acid test. Analytical Biochemistry 1978; 86: 271-278.
- Ellman GL. Tissue sulfhydryl groups. Archives of Biochemistry and Biophysics 1959; 82: 70-77.
- Tarpey M, Wink DA, Grisham MB. Methods for detection of reactive metabolites of oxygen and nitrogen: in vitro and in vivo considerations. The American Journal of Physiology-Regulatory Integrative and Comparative Physiology 2004; 286: R431-R444.
- Paglia DE, Valentine WN. Studies on the quantitative and qualitative characterization of erythrocyte glutathione peroxidase. J Lab Clin Med 1967; 70: 158-169.
- Nishikimi M, Appaji N, Yagi K. The occurrence of superoxide anion in the reaction of reduced phenazinemethosulfate and molecular oxygen.BiochemBiophys Res Commun1972; 46: 849-854.
- Aebi H. Catalase in vitro. Methods Enzymol1984; 105: 121-126.
- Bancroft J, Gamble. Theory and Practice of Histology Techniques. 6thEdChurchill Livingstone Elsevier 2008.
- Afifi NM, Hanon AF. Histological and immunohistochemical study on the possible cardioprotective role of acetylcysteine in oral formalin myocardial toxicity in adult albino rats. The Egyptian Journal of Histology 2011; 34: 859-869.
- Leslie K, Taatjes DJ, Schwarz J, vonTurkovich M, Lowt RB. Cardiac Myofibroblasts Express Alpha Smooth Muscle Actin during Right Ventricular Pressure Overload in the Rabbit. Am J Pathol 1991; 139: 207-216.
- Massoud AA, El-Atrash A, Tousson E, Ibrahim W, Abou-Harga H. Light and ultrastructural study in the propylthiouracil-induced hypothyroid rat heart ventricles and the ameliorating role of folic acid. Toxicology and Industrial Health 2012; 28: 262-270.
- Cakc-Milosevic M, Korac A, Davidovi V. Methimazole-Induced Hypothyroidism In Rats: Effects On Body Weight And Histological Characteristics Of Thyroid Gland. Jugoslov Med Biohem 2004; 23: 143-147.
- Klein I, Danzi S. Thyroid disease and the heart. Circulation 2007; 116: 1725-1735.
- Weber KT, Sun Y, Bhattacharya SK, Ahokas RA, Gerling IC. Myofibroblast mediated mechanisms of pathological remodelling of the heart. Nat Rev Cardiol 2012; 10: 15-26.
- Gosteli-Peter MA, Harder BA, Eppenberger HM, Zap fJ, Schaub MC. Triiodothyronine induces over-expression of alpha-smooth muscle actin, restricts myofibrillar expansion and is permissive for the action of basic fibroblast growth factor and insulin-like growth factor I in adult rat cardiomyocytes. J Clin Invest 1996; 98: 1737-1744.
- PaulinD, Huet A, Khanamyrian L. Desminopathies in muscle disease. J Pathol 2004; 204: 418-427.
- Capetanaki Y, Milner DJ, Weitzer G. Desmin in muscle formation and maintenance: Knockouts and consequences. Cell StructFunct 1997; 22: 103-116.
- Nagi MN, Mansour MA. Protective effect of thymoquinone against doxorubicin-inducedcardiotoxicity in rats: a possible mechanism of protection. Pharmacol Res 2000; 41: 283-289.
- Shariatifar A, Riazi M, Ebnolelm M, Jahromy MH. Effects of Nigella sativa L. Seed Extract on Fatigue, Blood Biochemical Parameters and Thyroid Function in Male Mice. Chinese Medicine 2014; 5: 16-21.
- Nazifi S, Mansourian M, NikahvalB, Razavi SM. The relationship between serum level of thyroid hormones, trace elements and antioxidant enzymes in dromedary camel (Camelusdromedarius). Trop Anim Health Prod 2009; 41: 129-134.
- Chattopadhyay S, Zaidi G, Das K, Chainy GBN. Effects of hypothyroidism induced by 6-n-propylthiouracil and its reversal by T3 on rat heart superoxide dismutase, catalase and lipid peroxidation. Indian J ExpBiol 2003; 41: 846-849.
- Choudhury S, Chainy GB, Mishro MM. Experimentally induced hypo- and hyper-thyroidism influence on the antioxidant defence system in adult rat testis. Andrologia2003; 35: 131-140.
- Petrulea MS, Duncea IH, Georgeta D, Gheorghe D, Nicoleta MA. Oxidative stress in experimental hypothyroidism: effect of vitamin e supplementation. Clujul Medical2010; 83: 245.
- Badary OA, Taha RA, Gamal el-din AM, Abdel-Wahab MH. Thymoquinone is a potent superoxide anion scavenger. Drug ChemToxicol 2003; 26: 87-98.
- Rassaf T, Poll LW, Brouzos P, Lauer T, Totzeck M, Kleinbongard P. Positive effects of nitric oxide on left ventricular function in humans. Eur Heart J 2006; 27: 1699-1705.
- Balligand JL, Cannon PJ. Nitric oxide synthases and cardiac muscle. Autocrine and paracrine influences. Arterioscler. ThrombVascBiol1997; 17: 1846-1858.
- Fellet AL, Arza P, Arreche N, Arranz C, Balaszczuk AM. Nitric oxide and thyroid gland: modulation of cardiovascular function in autonomic-blocked anaesthetized rats. ExpPhysiol 2004;89:303-312.
|